For the second part of the discussion, you will apply what you have learned from the course materials in this module. By Thursday, create and pos
For the second part of the discussion, you will apply what you have learned from the course materials in this module. By Thursday, create and post separately, two well-crafted questions for your classmates to answer. Each question must include at least 3 – 5 sentences of background information before the questions are asked. You must start each question post with “CLASSMATE QUESTION.” Please be sure to research your questions and add references in APA style format.
Below are the google slide PowerPoint for the chapter and I have also attached the chapter reading. I need 3 references for each question. YOU DO NOT NEED TO ANSWER THE QUESTIONS.
CHAPTER 18 Physiology of the Cardiovascular System
STUDENT LEARNING OBJECTIVES
At the completion of this chapter, you should be able to do the following:
1.List the basic components of the cardiovascular system.
2.Describe the structures of the heart and how they serve to pump blood.
3.Discuss the deflection waves of a normal ECG and explain their significance.
4.Outline the basic steps of the cardiac cycle.
5.Discuss the primary principle of circulation and the significance of high arterial blood pressure.
6.Describe factors that affect stroke volume and heart rate.
7.Discuss the significance of peripheral resistance in the cardiovascular system.
8.Discuss the significance of the vasomotor control mechanism.
9.Discuss how the respiratory pump and skeletal muscle pump work to assist the venous return of blood to the heart.
10.Outline the hormonal mechanisms that regulate blood volume.
11.Discuss the significance of the pulse mechanism.
12.List several major pulse points in the body.
LANGUAGE OF SCIENCE AND MEDICINE
Before reading the chapter, say each of these terms out loud. This will help you avoid stumbling over them as you read.
ADH mechanism (A-D-H MEK-ah-nih-zem)
[ADH antidiuretic hormone, mechan- machine, -ism state]
ANH mechanism (A-N-H MEK-ah-nih-zem)
[ANH atrial natriuretic hormone, mechan- machine, -ism state]
atrioventricular (AV) bundle (ay-tree-oh-ven-TRIK-yoo-lar BUN-del)
[atrio- entrance courtyard, -ventr- belly, -icul- little, -ar relating to]
atrioventricular (AV) node (ay-tree-oh-ven-TRIK-yoo-lar)
[atrio- entrance courtyard, -ventr- belly, -icul- little, -ar relating to, nod- knot]
baroreceptor (bar-oh-ree-SEP-tor)
[baro- pressure, -recept- receive, -or agent]
cardiac cycle (KAR-dee-ak SYE-kul)
[cardi- heart, -ac relating to, cycle circle]
cardiac output (CO) (KAR-dee-ak)
[cardi- heart, -ac relating to]
conduction system of the heart (kon-DUK-shen SIS-tem)
[conduct- lead, -tion process, system organized whole]
contractility (kon-trak-TIL-ih-tee)
[con- together, -tract- drag or draw, -il- of or like, -ity quality of]
diastole (dye-ASS-toh-lee)
[dia- through, -stole contraction]
diastolic blood pressure (dye-ah-STOL-ik PRESH-ur)
[dia- apart or through, -stol- contraction, -ic relating to]
ectopic pacemaker (ek-TOP-ik PAYS-may-ker)
[ec- out of, -top- place, -ic relating to]
electrocardiogram (ECG or EKG) (eh-lek-troh-KAR-dee-oh-gram)
[electro- electricity, -cardio- heart, -gram drawing]
heart murmur
[murmur hum]
heart rate (HR)
hemodynamics (hee-moh-dye-NAM-iks)
[hemo- blood, -dynami- force, -ic relating to]
peripheral resistance (peh-RIF-er-al)
[peri- around, -phera- boundary, -al relating to]
primary principle of circulation (PRY-mair-ee PRIN-sip-al of ser-kyoo-LAY-shun)
[prim- first, -ary relating to, princip- foundation, circulat- go around, -tion process]
pulse
[pulse beat]
pulse pressure (PRESH-ur)
[pulse beat]
pulse wave
[pulse beat]
P wave
[named for letter of Roman alphabet]
QRS complex (Q R S KOM-pleks)
[named for letters of Roman alphabet]
renin-angiotensin-aldosterone system (RAAS) (REE-nin-an-jee-oh-TEN-sin-al-DAH-stair-ohn SIS-tem)
[ren- kidney, -in substance, angio- vessel, -tens- pressure or stretch, -in substance, aldo- aldehyde, -stero- solid or steroid derivative, -one chemical, system organized whole]
residual volume (ree-ZID-yoo-al)
[residu- remainder, -al relating to]
sinoatrial (SA) node (sye-no-AY-tree-al)
[sin- hollow (sinus), -atri- entrance courtyard, -al relating to, nod- knot]
sphygmomanometer (sfig-moh-mah-NOM-eh-ter)
[sphygmo- pulse, -mano- thin, -meter measure]
stroke volume (SV)
subendocardial branch (sub-en-doh-KAR-dee-al)
[sub- under, -endo- within, -cardi- heart, -al relating to]
systole (SIS-toh-lee)
[systole contraction]
systolic blood pressure (sis-TOL-ik PRESH-ur)
[systole- contraction, -ic relating to]
T wave
[named for letter of Roman alphabet]
vasoconstriction (vay-soh-kon-STRIK-shun)
[vaso- vessel, -constrict- draw tight, -tion state]
vasodilation (vay-soh-dye-LAY-shun)
[vaso- vessel, -dilat- widen, -tion state]
vasomotor center (vay-so-MOH-tor)
[vaso- vessel, -motor move]
vasomotor mechanism (vay-so-MOH-tor MEK-ah-nih-zem)
[vaso- vessel, -motor move, mechan- machine, -ism state]
venous pump (VEE-nus pump)
[ven- vein, -ous relating to]
venous return (VEE-nus)
[ven- vein, -ous relating to]
BOBBY was in a hurry to finish the last job of the day. He had been called in to help complete and inspect the wiring in a museum that was due to open the next week. Bobby called to his coworker, Jerry, to confirm the current was off to the electrical box on which he was working. When he heard a positive response, he climbed the ladder and reached up to tighten a few loose screws. As he was tightening the screws with his right hand, he lost his balance and reached up with his left hand to catch hold of the ladder…but instead caught a live wire that sent a jolt of electricity through his body.
Jerry came running and knocked the ladder out from under Bobby so he would fall to the floor, breaking the electric arc. “Are you okay?” he asked Bobby, who appeared dazed, but was conscious. “I don't feel so great,” he replied, smiling weakly. Then he collapsed. Jerry yelled at the other workers in the room to call 911, checked Bobby's pulse, and started CPR. The foreman rushed in with an automated external defibrillator (AED) and attached the electrodes to Bobby's chest. The AED's mechanical voice said, “Press button when clear.”
As you read through this chapter, you'll understand the electrical mechanism that operates your heart.
Now that you have read this chapter, see if you can answer these questions about the shock Bobby received in the Introductory Story.
When activated, the AED shocks the heart—the intended purpose being electrical stimulation of the cardiac muscle cells to elicit a response from the heart's pacemaker that will cause the cells to contract in unison to effectively pump blood.
1.What bundle of cells in Bobby's heart (and yours) is known as the pacemaker?
a.SV node
b.SA node
c.AV node
d.AV bundle
When the paramedics arrive, they rush Bobby to the hospital. “Let's get an EKG!” the attending physician calls out.
2.What is an EKG?
a.Electrocardiogram
b.Electrocirculogram
c.Encephalocardiogram
d.Enhancercardiogram
3.Ventricular depolarization is shown as which part of the EKG?
a.V wave
b.P wave
c.T wave
d.QRS complex
To solve a case study, you may have to refer to the glossary or index, other chapters in this textbook, A&P Connect, Mechanisms of Disease, and other resources.
FUNCTION OF THE HEART AND BLOOD VESSELS
Our bodies are never at rest. In fact, a continuous supply of energy is required to maintain a relatively constant internal environment. This homeostatic maintenance of our body is due in large part to the continuous and controlled movement of blood throughout our circulatory system. Blood carries out most of its important transport functions in the thousands of miles of capillaries that comprise much of this system. As you might suspect, however, the body's total blood volume is not evenly distributed. The regulation of blood pressure and blood flow must therefore change in response to cellular activity.
There are many control mechanisms that help to regulate and integrate the diverse functions and component parts of our cardiovascular system. This system must work to supply blood to specific body areas according to their immediate needs. These mechanisms ensure a relatively constant internal environment surrounding each body cell. In this chapter we will explore the control mechanisms that regulate the pumping action of the heart. We will also see how these mechanisms ensure the smooth and directed flow of blood throughout our circulatory system.
HEMODYNAMICS
Hemodynamics refers to the various mechanisms that influence the movement of blood. This is vital, of course, because different organs may need vastly different amounts of blood flow, depending on their metabolic activity. For example, working muscles need a far greater blood supply than do resting muscles. This is because tissues and organs (such as working muscles) with a greater metabolic rate obviously need more oxygen. And that oxygen is delivered by the RBCs of flowing blood in the capillaries. Because the amount of blood in our bodies is relatively constant, this means that the flow of blood to specific areas must be managed according to their activities. We will begin with a discussion of the physiology of the heart and then examine blood flow throughout the cardiovascular system.
A&P CONNECT
Whether blood flows straight through vessels or in a swirling, turbulent pattern has great clinical significance. For example, turbulent flow may signal a partially blocked artery and may promote the formation of dangerous blood clots. Learn more about this concept in Focus on Turbulent Blood Flow online at A&P Connect.
THE HEART AS A PUMP
Recall from Chapter 17 that the left side of the heart services the systemic circulatory route, which supplies blood flow to the entire body, except to the lungs. The right side of the heart services the pulmonary circulatory route, which supplies blood flow to and from the lungs. Both routes require coordination so that they function as a single pumping structure. To do this, the impulses (action potentials) that trigger contraction must be coordinated. The heart must have a system to generate rhythmic impulses. It must then distribute these impulses to the different regions of the myocardium. This distribution is accomplished by the impulse-conducting pathway. Four structures make up the core of the conduction system of the heart:
1.Sinoatrial (SA) node
2.Atrioventricular (AV) node
3.AV bundle (bundle of His)
4.Subendocardial branches (Purkinje fibers)
You can see these structures represented in Figure 18-1. Please refer to this figure as you read through the following discussion.
The structures that make up the heart's conduction system are composed of cells that differ in function from those of ordinary cardiac muscle: The heart conduction cells cannot contract strongly. Instead, they permit the generation or rapid conduction of an action potential throughout the myocardium.
Normally, the cardiac impulses that control heart contraction begin in the sinoatrial (SA) node. This node, also called the “pacemaker,” is located just below the atrial epicardium at its junction with the superior vena cava (Figure 18-1, A). The pacemaker cells in the SA node have an intrinsic rhythm. This means that they do not require nervous input from the brain or spinal cord. They themselves initiate impulses at regular intervals. In fact, if you remove pacemaker cells and put them in a nutrient solution, they will continue to beat! They do not require nervous or hormonal stimulation to contract.
FIGURE 18-1 Conduction system of the heart. Specialized cardiac muscle cells (boldface type) in the wall of the heart rapidly initiate or conduct an electrical impulse throughout the myocardium. Both the sketch of the conduction system (A) and the flowchart (B) show the origin and path of conduction. The signal is initiated by the SA node (pacemaker) and spreads directly to the rest of the right atrial myocardium. From there, it travels to the left atrial myocardium by way of a bundle of interatrial conducting fibers, and then to the AV node by way of three internodal bundles. The AV node then initiates a signal that is conducted through the ventricular myocardium by way of the AV bundle (of His) and subendocardial branches (Purkinje fibers).
However, in a living heart, there are nervous and hormonal components that influence the cells of the pacemaker.
Here's an overview of how the heart's conduction system works (Figure 18-1, B). Each impulse generated at the sinoatrial (SA) node travels swiftly throughout the muscle fibers of both atria. (An interatrial bundle of conducting fibers provides rapid conduction to the left atrium.) Thus, the atria begin to contract. The action potential next enters the atrioventricular (AV) node via three internodal bundles. These bundles are also composed of conducting fibers. Here the conduction of the impulse slows considerably. This allows the atria to contract completely before the conduction reaches the ventricles below.
After passing slowly through the AV node, the conduction velocity increases again as the impulse is relayed through the atrioventricular (AV) bundle (also called the bundle of His). At this point, the right and left bundle branches and the subendocardial branches (also called the Purkinje fibers) in which they terminate conduct the impulses throughout the muscle of both ventricles. This impulse stimulates the ventricular muscle fibers to contract almost simultaneously.
Thus the SA node initiates each heartbeat and sets the basic pace—it is the heart's own natural pacemaker. Under the influence of autonomic and endocrine controls, the SA node will normally “fire” at an intrinsic rhythmical rate of 70 to 75 beats per minute under resting conditions. However, if for any reason the SA node loses its ability to generate an impulse, pacemaker activity will shift to another excitable component of the conduction system. These might include the AV node or the subendocardial branches. Pacemakers other than the SA node are abnormal. They are called ectopic pacemakers. Unfortunately, such ancillary pacemakers usually set a much slower rhythm—only 40 to 60 beats per minute. If the heart's own pacemaker fails to maintain a healthy heart rhythm, an artificial pacemaker can be implanted to restore normal function.
A&P CONNECT
Artificial pacemakers can help keep individuals with damaged hearts alive for many years. Check out Artificial Cardiac Pacemakers online at A&P Connect to see how these devices work and how they are implanted.
Electrocardiogram (ECG)
Electrocardiography
Impulse conduction in the heart generates tiny electrical currents that spread through surrounding tissues to the surface of the body. This fact has great clinical importance because these currents can be measured with an electrocardiograph. An electrocardiogram (ECG) is produced by attaching electrodes of a recording voltmeter (the electrocardiograph) to the chest and/or limbs of the subject (Figure 18-2, A).
FIGURE 18-2 Electrocardiogram. A, A nurse monitors a patient's ECG as he exercises on a treadmill. B, Idealized ECG deflections represent depolarization and repolarization of cardiac muscle tissue. C, Principal ECG intervals between P, QRS, and T waves. Note that the P-R interval is measured from the start of the P wave to the start of the Q wave.
FIGURE 18-3 The basic theory of electrocardiography.
FIGURE 18-4 Events represented by the electrocardiogram (ECG). It is impossible to illustrate the invisible, dynamic events of heart conduction in a few cartoon panels or “snapshots.” However, the sketches here give you an idea of what is happening in the heart as an ECG is recorded. Note that depolarization triggers contraction in the affected muscle tissue. Thus cardiac muscle contraction occurs after depolarization begins.
(The abbreviation for an electrocardiogram is ECG when it is written and EKG when it is spoken.) The ECG is not a record of the heart's contractions but of the electrical events that precede the contractions.
You can see changes in voltage (which represent changes in the heart's electrical activity) as deflections of the line in a recording voltmeter (Figure 18-3). We've simplified the situation somewhat by showing only a single cardiac muscle fiber with the two electrodes of a recording voltmeter nearby.
Before the action potential reaches either electrode, there is no difference in charge between them. Thus, no change in voltage is recorded on the voltmeter graph (Figure 18-3, step 1).
As an action potential reaches the first electrode, the external surface of the sarcolemma becomes relatively negative. This results in an upward deflection of the pen of the recording chart (Figure 18-3, step 2). (Note that the voltmeter records the difference in charge between the two electrodes.) When the action potential also reaches the second electrode, the pen returns to the zero baseline. This happens because there is no difference in charge once again between the two electrodes (Figure 18-3, step 3).
As the end of the action potential passes the first electrode, the sarcolemma is again relatively positive on its outer surface. This causes the pen to again deflect away from the baseline. This time, however, because the direction of the negative and positive electrodes is reversed, the pen deflects downward (Figure 18-3, step 4). After the end of the action potential also passes the second electrode, the pen again returns to the zero baseline (Figure 18-3, step 5).
In short, when ECG electrodes are set up this way, depolarization of the cardiac muscle causes a deflection upward; repolarization causes a deflection downward. However, depending on the location of electrodes relative to heart muscle, the direction of ECG waves can vary. It is this activity that is detected in an electrocardiogram. Many ECG setups in use today show these voltage fluctuations in real time on a video monitor and at the same time records them in a computer file and on a paper chart (like the one represented in Figure 18-3).
ECG Waves
We will limit our discussion of electrocardiography to normal ECG deflection waves and the ECG intervals between them. As we do so, please refer to Figure 18-2 and the sequence of events presented in Figure 18-4.
As you can see in Figures 18-2 and 18-4, the normal ECG is composed of deflection waves called the P wave, QRS complex, and T wave. (The letters do not represent any words; they were simply chosen as an arbitrary sequence of letters!)
The P wave represents depolarization of the atria. It measures the deflection caused by the passage of an electrical impulse from the SA node through the musculature of the atria.
The QRS complex represents the depolarization of the ventricles. This is a multi-step process. First there is the depolarization of the interventricular septum. This is followed by the spread of depolarization by the subendocardial branches (Purkinje fibers) through the lateral ventricular walls. All three deflections, then—Q, R, and S together—represent the entire process of depolarization of the ventricles.
There is a slight catch. At the same time that the ventricles are depolarizing, the atria are repolarizing. You might expect to see some indication of this in the ECG tracing. However, the massive ventricular depolarization that is occurring at the same time literally “drowns out” the relatively small voltage fluctuation produced by atrial repolarization. For this reason, the QRS complex represents the net deflection due to both ventricular depolarization and atrial repolarization.
The T wave reflects repolarization of the ventricles. Depending on exactly where the electrodes are placed relative to the direction of electrical activity in the ventricular myocardium, this repolarization wave may deflect the ECG trace in the same direction as in depolarization.
ECG Intervals
The principal ECG intervals between P, QRS, and T waves are illustrated for you in Figure 18-2, C. Measurement of these intervals can provide important information concerning the rate of conduction of an action potential through the heart.
1. What is meant by hemodynamics?
2. Briefly outline the conduction system of the heart.
3. How does an electrocardiograph work?
4. What are the major deflections in an ECG?
5. What conduction event does each type of ECG wave represent?
Cardiac Cycle
The cardiac cycle describes a complete heartbeat or a single pumping cycle. It consists of contraction (systole) and relaxation (diastole) of both atria and both ventricles. First, the two atria contract simultaneously. Then, as the atria relax, the two ventricles contract and relax. As a result, all the chambers of the heart do not contract as a single unit. This alternation of contraction and relaxation imparts a pumping rhythm to the heart.
During the following discussion, please refer often to Figure 18-5, which shows the major phases of the cardiac cycle.
Atrial Systole
The contracting myocardium of the atria forces the blood into the ventricles below. The atrioventricular (cuspid) valves are opened during this phase to allow for the passage of blood into the relaxed ventricles. The semilunar valves are closed, preventing blood return from the aorta or the pulmonary trunk. This part of the cycle is represented by the P wave on an ECG. Passage of the electrical wave of depolarization is then followed almost immediately by actual contraction of the atrial musculature.
Ventricular Contraction
During the brief period of ventricular contraction (between the start of ventricular systole and the opening of the semilunar valves), the volume of blood in the ventricles remains constant (isovolumetric). However, the pressure inside the ventricles increases rapidly. The ventricular systole is marked by the R wave on the ECG. At this time, the first audible heart sound (often described as a “lubb”) is produced.
Ejection
The semilunar valves open and blood is ejected under great force from the ventricles. At this point, the pressure in the ventricles exceeds the pressure in the pulmonary artery and aorta, and blood is pushed into these vessels. This rapid ejection is characterized by a marked increase in ventricular and aortic pressure. The T wave of the ECG appears during the later, long phase of reduced ejection. You might think of this as the tail end of the contraction. However, a considerable quantity of blood, called the residual volume, remains in the ventricles even at the end of the ejection period. In heart failure, the residual volume remaining in the ventricles may greatly exceed the volume ejected into the aorta and pulmonary trunk.
Ventricular Relaxation
Diastole—the relaxation of the ventricles—begins with this period between closing of the semilunar valves and the opening of the atrioventricular valves. At the end of ventricular contraction, the semilunar valves close so that blood cannot re-enter back into the ventricles from the great vessels. The second heart sound (described as a “dupp”) is now heard.
The atrioventricular valves do not open until the pressure in the atrial chambers exceeds the pressure in the relaxed ventricles. The result is a dramatic fall in intraventricular pressure—but no change in blood volume. This is an isovolumetric phase—both sets of valves are closed.
Passive Ventricular Filling
The continuing return of venous blood from the venae cavae and the pulmonary veins increases pressure within both atria until the atrioventricular valves are forced open. When this happens, blood rushes into the relaxed ventricles. This rapid influx of blood lasts only about 0.1 second but results in a
FIGURE 18-5 The cardiac cycle. The five steps of the heart's pumping cycle described in the text are shown as a series of changes in the heart wall and valves. The term isovolumetric means that the volume remains constant.
dramatic increase in the volume of blood in the ventricle. The abrupt inflow of blood that occurs immediately after opening of the AV valves is followed by a slow and continuous flow of venous blood into the atria. This blood then flows through the open AV valves and into the ventricles, slowly building up the blood pressure and volume within the ventricles.
Heart Sounds
The first “lubb” or systolic sound is caused largely by the contraction of the ventricles and by the closing atrioventricular valves. It is longer and lower than the second or diastolic sound, which is short and sharp. Vibrations of the closing semilunar valves cause this second “dupp” sound.
Heart sounds have clinical significance and can provide information about the valves. Any variation from normal “lubb-dupp” sounds may suggest imperfect valve function. A heart murmur is one commonly heard type of abnormal heart sound. Sometimes it is described as a “swishing” sound. This may indicate an incomplete closing of the valves or stenosis (constriction or narrowing) of the valves.
6. Briefly outline the steps of the cardiac cycle.
7. When are heart sounds of medical importance?
PRIMARY PRINCIPLE OF CIRCULATION
In order for blood to flow within the circulatory system, there must be a gradient from high pressure to low pressure (Figure 18-6). This is sometimes called the primary principle of circulation. For example, blood enters an arteriole at 85 mm Hg and leaves at 35 mm Hg. The blood thus moves down a pressure gradient (from 85 mm Hg to 35 mm Hg) as it flows through the arteriole. The pressure difference drives the flow of blood.
ARTERIAL BLOOD PRESSURE
High pressure in the arteries must be maintained to keep blood flowing through the circulatory system. The volume of blood within the arteries largely determines arterial blood pressure. Thus, an increase in arterial blood volume tends to increase arterial pressure. Likewise, a decrease in arterial volume tends to decrease arterial pressure. However, many factors determine arterial pressure through their influence on arterial volume. Two of the most important, cardiac output and peripheral resistance, are directly proportional to blood volume, as we will see below.
FIGURE 18-6 The primary principle of circulation. Fluid always travels from an area of high pressure to an area of low pressure. Water flows from an area of high pressure in the tank (100 mm Hg) toward the area of low pressure above the bucket (0 mm Hg). Blood tends to move from an area of high average pressure at the beginning of the aorta (100 mm Hg) toward the area of lowest pressure at the end of the venae cavae (0 mm Hg). Blood flow between any two points in the circulatory system can always be predicted by the pressure gradient.
Cardiac Output
Cardiac output (CO) is the amount of blood that flows out of a ventricle per unit of time. For example, the resting cardiac output from the left ventricle into the systemic arteries is about 5,000 ml/min. The cardiac output influences the flow rate to the various organs of the body. For the sake of our discussion, we will focus on the cardiac output from the left ventricle into the systemic loop.
Cardiac output is determined by the volume of blood pumped out of a ventricle by each beat (stroke volume or SV) and by heart rate (HR). Because contraction of the heart is called systole, sometimes the volume of blood pumped by one contraction is called the systolic discharge. Stroke volume, or volume pumped per heartbeat, is one of two major factors that determine cardiac output. CO can be determined by the following equation:
SV
(
volume
/
beat
)
×
HR
(
beats
/
min
)
=
CO
(
volume
/
min
)
Thus the greater the stroke volume, the greater the CO (but only if the heart rate remains constant). Anything that changes the rate of the heartbeat or its stroke volume tends to change CO. This means that anything that makes the heart beat faster or stronger (increases its stroke volume) tends to increase CO and therefore arterial blood volume and pressure. Conversely, anything that causes the heart to beat more slowly or more weakly (decreases its stroke volume) tends to decrease CO, arterial volume, and blood pressure.
The following sections, and Figure 18-7, summarize a few of the major factors that affect cardiac output.
Factors that Affect Stroke Volume
Mechanical, neural, and chemical factors regulate the strength of the heartbeat and therefore its stroke volume.
One mechanical factor that helps determine stroke volume is the length of the myocardial fibers at the beginning of ventricular contraction. According to the Frank-Starling mechanism, the longer or more stretched the heart fibers are at the beginning of contraction (up to a critical limit), the stronger is their contraction. The more blood returned to the heart per minute, the more stretched will be their fibers. This will lead to stronger contractions and a larger volume of blood ejected with each contraction.
However, if too much blood stretches the heart beyond a certain critical limit, the myocardial muscle seems to lose its elasticity. As a result the heart contracts less vigorously.
We can now see that the heart pumps out what it receives. That is, within certain limits, the strength of myocardial contraction matches the pumping load. This is contrary to most mechanical pumps that do not adjust themselves to their input with every stroke. In the case of a human heart, under ordinary conditions, it automatically adjusts output (stroke volume) to input (venous return to the heart).
Other factors that influence stroke volume are neural and endocrine chemical factors. Norepinephrine (released by sympathetic fibers in the cardiac nerve) and epinephrine (released into the blood by the adrenal medulla) can both increase the strength of contraction, or contractility, of the myocardium. This increased contractility of the heart muscle forces more blood volume out of the heart per cardiac stroke, thus increasing the stroke volume.
Factors that Affect Heart Rate
Although the sinoatrial node normally initiates each heartbeat, the heart rate it sets can be altered. In fact, various factors can and do change the rate of the heartbeat. One major modifier is the ratio of sympathetic and parasympathetic impulses conducted to the node per minute. This is because autonomic control of heart rate is the result of opposing influences between the parasympathetic (chiefly vagus) and sympathetic (cardiac nerve) stimulation. The parasympathetic stimulation is inhibitory—mediated by acetylcholine released by the vagus nerve. The sympathetic stimulation is stimulatory—mediated by the release of norepinephrine at the distal end of the cardiac nerve.
Cardiac Pressoreflexes
Receptors sensitive to changes in pressure (baroreceptors) are located in two places near the heart. The aortic baroreceptors and the carotid baroreceptors send afferent nerve fibers to cardiac control centers in the medulla oblongata. These stretch
FIGURE 18-7 Factors affecting cardiac output.
receptors, located in the aorta and carotid sinus, respectively, are vitally important to controlling heart rate. Baroreceptors
Collepals.com Plagiarism Free Papers
Are you looking for custom essay writing service or even dissertation writing services? Just request for our write my paper service, and we'll match you with the best essay writer in your subject! With an exceptional team of professional academic experts in a wide range of subjects, we can guarantee you an unrivaled quality of custom-written papers.
Get ZERO PLAGIARISM, HUMAN WRITTEN ESSAYS
Why Hire Collepals.com writers to do your paper?
Quality- We are experienced and have access to ample research materials.
We write plagiarism Free Content
Confidential- We never share or sell your personal information to third parties.
Support-Chat with us today! We are always waiting to answer all your questions.
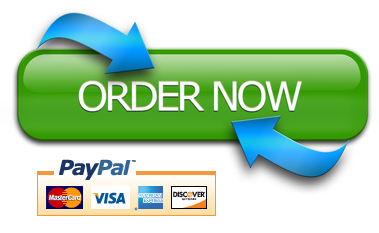