Write a critique on the aging paper (between 750 to 900 words excluding the title page) I have to read the paper and critique it. Talk about the pap
Write a critique on the aging paper (between 750 to 900 words excluding the title page)
I have to read the paper and critique it. Talk about the paper and the results and what the graphs mean. Also talk about what could've been done better.
Naked mole-rat has increased translational fidelity compared with the mouse, as well as a unique 28S ribosomal RNA cleavage Jorge Azpuruaa,1, Zhonghe Kea,1, Iris X. Chena, Quanwei Zhangb, Dmitri N. Ermolenkoc, Zhengdong D. Zhangb, Vera Gorbunovaa,2, and Andrei Seluanova,2
aDepartment of Biology, University of Rochester, Rochester, NY 14627; bDepartment of Genetics, Albert Einstein College of Medicine, New York, NY 10461; and cDepartment of Biochemistry and Biophysics, University of Rochester, Rochester, NY 14642
Edited* by Eviatar Nevo, Institute of Evolution, Haifa, Israel, and approved September 3, 2013 (received for review July 18, 2013)
The naked mole-rat (Heterocephalus glaber) is a subterranean euso- cial rodent with a markedly long lifespan and resistance to tumor- igenesis. Multiple data implicate modulation of protein translation in longevity. Here we report that 28S ribosomal RNA (rRNA) of the naked mole-rat is processed into two smaller fragments of un- equal size. The two breakpoints are located in the 28S rRNA di- vergent region 6 and excise a fragment of 263 nt. The excised fragment is unique to the naked mole-rat rRNA and does not show homology to other genomic regions. Because this hidden break site could alter ribosome structure, we investigated whether trans- lation rate and amino acid incorporation fidelity were altered. We report that naked mole-rat fibroblasts have significantly increased translational fidelity despite having comparable translation rates with mouse fibroblasts. Although we cannot directly test whether the unique 28S rRNA structure contributes to the increased fidelity of translation, we speculate that it may change the folding or dynamics of the large ribosomal subunit, altering the rate of GTP hydrolysis and/or interaction of the large subunit with tRNA dur- ing accommodation, thus affecting the fidelity of protein synthe- sis. In summary, our results show that naked mole-rat cells produce fewer aberrant proteins, supporting the hypothesis that the more stable proteome of the naked mole-rat contributes to its longevity.
aging | NMR
Naked mole-rats (NMRs; Heterocephalus glaber) are extremelongevity outliers compared with other rodents of their size. Although only slightly larger than a laboratory mouse, the NMR lives an order of magnitude longer, with a maximum recorded lifespan of more than 30 y for both reproductive and non- reproductive castes (1). It was also noted that NMRs are markedly resistant to the increased frailty that accompanies ag- ing in most metazoans. Furthermore, despite observations of many aged individuals, no neoplasia has been reported in the NMR (2, 3). NMR cells have been experimentally shown to be much more
resistant to oncogenic transformation than mouse cells (4, 5). The mechanism of tumor resistance is mediated by hypersensi- tivity of NMR cells to contact inhibition triggered by hyaluronan of extremely high molecular mass (5–7). Although these studies explain why the animals are tumor-resistant, they do not explain their overall longevity and their ability to maintain somatic in- tegrity until extreme age. Previous studies have indicated that the NMR is able to tolerate high intracellular levels of oxidized proteins and oxidized lipids (8–10). Furthermore, the NMR is notably able to maintain proteomic integrity (as evidenced by lower protein unfolding after treatment with urea) until very late in its lifespan without an age-dependent increase in protein ubiquitination, such as observed in short-lived mice (11). Multiple signaling pathways implicated in aging and cancer
converge on factors that modify the rate of translation initiation and elongation (12–15). One of the main functions of the mTOR
pathway is the promotion of protein translation in response to nutrients. Modulating the mTOR pathway genetically or phar- macologically, using the inhibitor rapamycin, has been shown to extend lifespan in yeast, Caenorhabditis elegans, Drosophila, and mice (14). The exact mechanism by which modulating protein translation via inhibition of mTOR extends lifespan is unclear. Two major explanations that were proposed are global reduction in mRNA translation, leading to better maintenance of protein homeostasis, or differential translation of specific mRNAs ben- eficial for longevity and stress resistance (reviewed in ref. 13). Proper protein folding and stability are heavily implicated in
aging. Overexpression of heat shock transcription factors and chaperones that aid protein folding can extend lifespan in Dro- sophila melanogaster (16) and C. elegans (17, 18). In bacteria, aging occurs by asymmetrical segregation of protein aggregates (19), and a similar mechanism has been described in yeast (20). The formation of aggregates as a result of mistranslated poly- peptides has been implicated in several aging-related diseases such as amyotrophic lateral sclerosis, Huntington’s disease, and Alzheimer’s disease (21). A major factor determining proteome quality is translational fidelity of the ribosome. Severe disruptions of translational fidelity have been shown to cause accumulation of aggregates and pathological neurodegeneration (22). The connection between translation and aging led us to ex-
amine NMR ribosomes for longevity-promoting phenotypes such as altered translation rate and translational fidelity. We describe a unique ribosomal DNA insertion, which is processed after rRNA transcription and breaks the NMR 28S rRNA into two distinct fragments. We assayed ribosomal translation rate (com- bined initiation and elongation) by measuring bulk incorporation
Significance
Molecular mechanisms responsible for differences in longevity between animal species are largely unknown. Here we show that the longest-lived rodent, the naked mole-rat, has more accurate protein translation than the mouse. Furthermore, we show that the naked mole-rat has a unique fragmented ribo- somal RNA structure. Such cleaved ribosomal RNA has been reported for only one other species of mammal. This article suggests the importance of protein translation in aging and provides insight into the mechanisms of longevity.
Author contributions: J.A., Z.K., Z.D.Z., V.G., and A.S. designed research; J.A., Z.K., I.X.C., Q.Z., and Z.D.Z. performed research; J.A., Z.K., Q.Z., D.N.E., Z.D.Z., V.G., and A.S. analyzed data; and J.A., Z.K., Z.D.Z., V.G., and A.S. wrote the paper.
The authors declare no conflict of interest.
*This Direct Submission article had a prearranged editor. 1J.A. and Z.K. contributed equally to this work. 2To whom correspondence may be addressed. E-mail: [email protected] or [email protected]
This article contains supporting information online at www.pnas.org/lookup/suppl/doi:10. 1073/pnas.1313473110/-/DCSupplemental.
17350–17355 | PNAS | October 22, 2013 | vol. 110 | no. 43 www.pnas.org/cgi/doi/10.1073/pnas.1313473110
D ow
nl oa
de d
fr om
h tt
ps :/
/w w
w .p
na s.
or g
by 7
3. 20
3. 24
5. 0
on A
pr il
1 2,
2 02
2 fr
om I
P a
dd re
ss 7
3. 20
3. 24
5. 0.
of [35S]-cystein/methionine into nascent polypeptide chains. We found that NMR and mouse skin fibroblasts have roughly equal rates of translation. By using firefly luciferase reporters with a variety of mutations, including amino acid substitutions or stop codons that required misincorporation or frameshift to occur to reactivate the luciferase, we found that primary NMR fibroblasts have a fourfold lower rate of amino acid misincorporation than primary mouse fibroblasts. These results suggest that NMR cells produce fewer aberrant proteins and support the hypothesis that the NMR’s stable proteome is a contributor to its longevity.
Results NMR 28S rRNA Has an Atypical Pattern. During routine extraction of RNA, we noticed that the 28S ribosomal RNA of the NMR was processed into two fragments of unequal size. NMR RNA extracts from several tissues (liver, lung, brain, spleen, kidney, and testis) exhibited an unusual rRNA band pattern, whereas mouse RNA extracts did not (Fig. 1A). We observed this pattern after using two different methods of RNA extraction in extracts prepared from tissues and cultured cell lines (Fig. S1A). We also extracted RNA from other nonmodel rodents (Fig. S1B) and found the break to be unique for the NMR. To confirm that the break was not a result of RNase
contamination, we combined NMR cells with mouse cells and simultaneously extracted RNA after homogenizing them. When independently extracted, the fibroblast RNA showed three bands and two bands for NMR and mouse, respectively. In the mixed sample, all bands could be seen, indicating that no nuclease contamination was cleaving the mouse rRNA (Fig. 1B). This NMR 28S rDNA was undergoing specific processing in vivo.
Mapping of the 28S rRNA Cleavage Sites. To determine the site and sequence of the NMR 28S rRNA breakage point, we first designed primers to conserved 28S rDNA sequence from the mouse genome. We estimated the location of the breakpoint region by noting that the sizes of the two fragments (2.5 and
3 kb) were only slightly different, meaning the break would be within ∼1 kb of the center. This allowed us to amplify a central 1-kb fragment of genomic NMR 28S rDNA. This fragment included 28S divergent region 6 (D6) that exhibited a 118-nt unique insertion compared with the mouse sequence flanked by regions of weak homology with the mouse D6 (Fig. 2). Using the 1-kb sequence and a publically available NMR coding DNA sequence, we designed primers to perform 5′ RACE and 3′ RACE. After aligning with the NMR 28S rDNA sequence, we identified the two cleavage sites and found that a 263-nt fragment flanked by 5-nt direct repeats 5′-CGGAC-3′ was cleaved out in the 28S rRNA, leaving behind the fragmented 28S rRNA. The 263-nt fragment included the 118-nt insertion that was not homologous to the mouse sequence (Fig. 2). The ribosomal DNA loci are repeated thousands of times, and from our RNA extractions, most of the products seemed to be cleaved. We used a bioinformatics approach to test whether the identified cut sites were conserved among all of the 28S rDNA loci in the NMR. From whole-genome shotgun reads from two different NMR genome sequences [Broad Institute (http://www.ncbi.nlm. nih.gov/nuccore/AHKG00000000) and Kim et al. (23)], the cut sites were found to be conserved among all of the rDNA loci (Fig. S2 and Table S1), which confirmed that the majority of 28S rRNAs are cleaved in the NMR.
NMR Translation Rate Does Not Differ from the Mouse. We hy- pothesized that the fragmented 28S rRNA might cause alternate assembly or structure of the NMR ribosome, with detectable consequences for protein synthesis rate or fidelity. To investigate whether the NMR ribosome had a different translation rate than
A
Mouse NMR Mix B
Naked Mole-Rat
28S
18S
Li ve
r Lu
ng
B ra
in
Sp le
en
K id
ne y
Te st
es
Li ve
r
Mouse
18S
28S cleaved
Fig. 1. NMR rRNA shows an unusual fragmented pattern. (A) 28R rRNA from multiple organs of the NMR appears as two fragments. Arrows indicate 28S fragments and an 18S band. (B) The fragmentation of NMR 28S rRNA is not caused by degradation during RNA extraction. Either NMR and mouse RNA were extracted from fibroblasts independently or the cells from both species were mixed together before extraction. RNA was separated on 1% denaturing agarose gel.
D6 region
Mouse 28S rRNA
Naked mole-rat 28S rRNA
Ctenomys 28S rRNA
A
B
154 bp
No break
Mouse
NMR
Mouse
NMR
Mouse
NMR
Mouse
NMR
296 bp
263 bp
255 bp
1864 1931
1965
2036
1932
1967
Fig. 2. Structure of the NMR 28S rRNA and localization of break sites. (A) Overall structures of the 28S rRNA in mouse, NMR, and Ctenomys. The mouse 28S rRNA has no cleavage sites. The D6 region of the NMR 28S rRNA contains a unique 118-nt insertion (shaded box). The two cleavage sites then excise a 263-nt fragment. Ctenomys is the only other known mammal with fragmented rRNA. The D6 region of the Ctenomys 28S rRNA contains a unique 106-bp insertion (shaded box) with the cleavage site located within this unique sequence. Thick black lines represent the 28S rRNA, the white box represents the D6 region, the arrows denote the cleavage sites, and the size of the D6 region and the distance between the two cleavage sites are indicated. (B) The alignment of the NMR and mouse 28S rDNA sequences. The box highlights the D6 region, the arrows indicate the cleavage sites, and hyphens indicate the gap.
Azpurua et al. PNAS | October 22, 2013 | vol. 110 | no. 43 | 17351
C EL L B IO LO
G Y
D ow
nl oa
de d
fr om
h tt
ps :/
/w w
w .p
na s.
or g
by 7
3. 20
3. 24
5. 0
on A
pr il
1 2,
2 02
2 fr
om I
P a
dd re
ss 7
3. 20
3. 24
5. 0.
the mouse ribosome, we implemented an assay based on mea- suring [35S]-cystein/methionine incorporation into nascent pro- tein. As the physiological temperature of the NMR is 32 °C, rather than 37 °C, and NMR fibroblasts are usually cultured at 32 °C, we tested the translation rate at both temperatures for both mouse and NMR cells. Mouse and NMR fibroblasts were given a pulse of [35S]-cystein/methionine for 30 min, cultured at 32 °C and 37 °C, total cell proteins were extracted, and the amount of radioactive isotope incorporation was measured by a scintillation counter. The translation rate was found to be altered twofold by shifting
the temperature by 5° in both species (Student t test, NMR, P = 0.02; mouse, P = 0.0027), but no significant differences were seen between the NMR and mouse cells when they were temperature- matched (Fig. 3A). To confirm that the protein quantification was accurate and that mouse and NMR fibroblasts have similar amounts of ribosomes, we performed Western blots on ribosomal protein S6 (RPS6). No large differences were seen in the overall amount of ribosomal protein or loading controls from mouse and NMR fibroblasts (Fig. 3B). Taken together, these results show that the NMR translation rate is equivalent to that of the mouse when they are temperature-matched, and that the cells express similar levels of a ribosomal protein.
NMR Has Higher Translational Fidelity than the Mouse. We next ex- amined whether altered ribosomal structure might affect the overall translation fidelity of proteins. To assay mouse and NMR cells for translational fidelity, we constructed a set of firefly lu- ciferase mutant reporters. In these reporters, a key amino acid in the luciferase protein (lysine 529) was mutated to completely destroy luciferase activity (24), and only if the wrong amino acid were incorporated into the nascent polypeptide would enzymatic activity be restored. The reporters included all triplet positions,
premature stop (third codon mutation at the beginning of the protein), and both positive and negative frameshifts by nucleo- tide insertion and deletion, respectively. The luciferase assay indicated that NMR cells had higher
protein translation fidelity than mouse cells. This was particularly noticeable when mouse and NMR cells were grown at 32 °C, as the NMR cells had ∼10-fold lower misincorporation frequency for the lysine triplet mutants, and these differences were highly statistically significant for all reporters (Student t test, P < 0.005) (Fig. 4A). At 37 °C, NMR cells had approximately a fourfold lower misincorporation; this was also statistically significant for all reporters (Student t test, P < 0.05) (Fig. 4B). Notably, the NMR cells were able to maintain a high trans-
lational fidelity even when forced to grow more quickly. We in- tegrated simian virus 40 (SV40) Large T-antigen (LargeT) and human Ras Glycine 12 to Valine (H-RasV12) mutant protein into wild-type NMR fibroblasts to induce more rapid growth via constant Ras signaling and abrogation of the p53/retinoblastoma protein pRB tumor suppressor pathways to prevent oncogene- induced senescence. The fidelity assays were then repeated on these cells grown at 32 °C and 37 °C. The translation error rate was not significantly different from in the primary NMR fibroblasts at either temperature (Fig. 4 A and B), indicating that even when NMR cells proliferate at a similar rate as mouse cells, they still maintained higher translational fidelity. This result suggests the NMR ribosomes are intrinsically more accurate than the mouse ribosomes.
Discussion Our study shows an unexpected processing of NMR 28S rRNA into two molecules of 2.5 and 3 kb, corresponding to 3′ and 5′ segments of the rRNA gene. The break occurs within the D6 region and results from two cuts that excise a 263-nt fragment containing a unique 118-nt sequence. This 118-nt sequence bears no significant homology to other known sequences. The cleavage occurs in all NMR organs that we analyzed, as well as in the primary fibroblast cultures. The excised fragment is flanked by 5-nt direct repeats, which
supports that the insert originated from a transposable element. The sequences distal to the cut sites share weak homology with the mouse D6, suggesting that the insert could have also been introduced by homologous recombination. We do not know the mechanism responsible for the cleavage of the NMR 28S rRNA. The cuts could be generated by a ribozyme activity residing within the D6 sequence itself, as was reported for R2 trans- posons residing within the rDNA locus (25). We tested this possibility by in vitro transcribing 28S rRNA; however, the in vitro transcribed 28S rRNA molecules remained uncleaved. Fi- nally, the cleavage may be mediated by a ribonuclease that rec- ognizes the D6 region. Fragmented rRNA has been found in Salmonella (26), pro-
tozoa (27, 28), a worm (29), and several arthropods (ref. 30 and references therein). However, in vertebrates there is only one other example of a fragmented rRNA, found in rodents of the genus Ctenomys, also known as tuco-tuco (31). In Ctenomys, 28S rRNA is cleaved within the D6 region, but the cleavage occurs with only a single cut site, and sequences surrounding the break share no homology with the NMR sequence (Fig. 2A). Interestingly, Cten- omys is a South American rodent that is phylogenetically distant from the NMR but shares similar ecology. Ctenomys is a sub- terranean rodent, with some species of the genus leading a social lifestyle. The maximum lifespan in captivity is not determined for Ctenomys, but in the wild, Ctenomys is short-lived, with an average lifespan of 3 y (32). We found that NMR cells had equivalent translation rates
(adjusted for physiological temperature). However, by construct- ing a set of unique luciferase reporters, we observed that NMR cells have substantially higher translation fidelity than mouse cells,
RPS6
-actin
NMR Mouse
p = 0.02
p = 0.0027
B
0
5
10
15
20
25
Tr an
sl at
io n
ra te
, C
P M
/ μg
p ro
te in
1,
00 0
NMR SF 32oC
NMR SF 37oC
MSF 32oC
MSF 37oC
A
Fig. 3. NMR and mouse have similar translation rates. (A) [35S]-cystein/ methionine incorporation assay performed on growing fibroblasts showing NMR translation occurs at roughly equal speed to that of mouse fibroblasts. The assays were performed at 32 °C and 37 °C for both mouse and NMR cells. NMR SF are primary NMR skin fibroblast and MSF are primary mouse skin fibroblast. (B) Western blot against RPS6, a protein component of the ma- ture ribosome (Upper) and β-actin as loading control (Lower).
17352 | www.pnas.org/cgi/doi/10.1073/pnas.1313473110 Azpurua et al.
D ow
nl oa
de d
fr om
h tt
ps :/
/w w
w .p
na s.
or g
by 7
3. 20
3. 24
5. 0
on A
pr il
1 2,
2 02
2 fr
om I
P a
dd re
ss 7
3. 20
3. 24
5. 0.
even when transformed with oncogenes to increase their cell cycle speed. NMR cells also displayed higher fidelity of translation for all errors we tested, including misincorporation, stop codon skip- ping, and frameshifts. A potential caveat of our translation fidelity assay is that active firefly luciferase could be generated not only by incorrect translation but also by incorrect transcription or random mutations of the plasmid DNA during cell growth. This seems unlikely given that if replication or transcription produced active luciferase, we would not see the expected nucleotide position bias that the assay clearly shows (with the third position being the least mistranslated). Furthermore, we would not expect to see such a large bias in favor of insertions over deletions. The mis- incorporation rate we detect in our assay is also between 1:1,000 and 1:10,000, which is consistent with reports in the literature (24). Thus, we conclude that most of the luminescence from our assay comes from mistranslation, rather than from mistakes at the nucleotide level. Pérez et al. reported that the NMR proteome has higher levels
of cysteine than the mouse proteome and that the proteins are resistant to unfolding stressors such as 1 M urea. These results are consistent with high levels of translation fidelity (11). According to our results, the mouse proteome would have two- to 10-fold higher levels of proteins with misincorporated amino acids relative to the NMR. These misincorporated amino acids could increase the number of proteins that are unstable under challenging conditions, such as urea treatment. Pérez et al. also report that the NMR does not show an age-dependent increase in protein ubiquitination. This is consistent with the high fidelity of protein translation in the NMR. More recently, it has been reported that NMR proteins maintain a higher level of activity after carbonylation and oxidation than the equivalent mouse proteins (33). Again, it is possible that fewer amino acid mis- incorporations allow proteins to tolerate more stochastic improper modifications and oxidative damage, and thus retain tertiary struc- ture and activity. Given the remarkable longevity of the NMR, it is possible that
increased translational fidelity plays a role in its longevity. The “error catastrophe theory of aging” first proposed by Orgel in 1963 (34) and explored in Escherichia coli by Edelmann and Gallant in 1977 posits that early defects in translational fidelity lead to a vicious cycle of progressively more severe defects in protein homeostasis (35). Differences in translational fidelity may play an important role in determining lifespan. Many age- related disorders are caused by protein aggregation (21), and problems with translational fidelity can lead to the accumulation of misfolded protein and the formation of inclusion bodies (22). The proteome is maintained by various mechanisms, all of which show an age-dependent decline (36). During heat stress, chaper- ones are up-regulated, but this mechanism fails with age (37, 38). Proteasome activity also declines with age (39). Aging also leads to a decline in macroautophagy, which eliminates misfolded or in- correctly glycosylated proteins from the cell (40). It is possible that when proteome maintenance mechanisms fail, it allows the aberrant mistranslated proteins to accumulate. Species, such as the NMR, with a lower overall level of mistranslated proteins would thus be buffered against some of the decline of old age, given that they have fewer misfolded proteins to begin with. The main source of mistranslated proteins in E. coli is discrimi-
nation of noncognate or near-cognate tRNAs during translation (24). The ribosome structure itself confers at least some of the fidelity of the process, as shown by hyperaccurate ribosomes (41), and its sequence and structure (42) may be altered by natural se- lection in species that evolve long lifespans. Indeed, in E. coli, there is an observed evolutionary tradeoff between ribosomal speed and accuracy (41). Because the NMR in vitro translation system cur- rently cannot be reconstituted from purified components, we are unable to directly test whether 28S cleavage is responsible for im- proved translation fidelity in the NMR. The cleavage leads to
A
WT Control 1st 2nd 3rd Stop FS+ FS-
Firefly Luciferase Reporter
Fi re
fly /
R en
ill a
Lu m
in es
ce nc
e
0
0.01
0.02
0.03
0.04
5
10
15
20
* *
* * *
*
B
WT Control 1st 2nd 3rd Stop FS+ FS-
Firefly Luciferase Reporter
Fi re
fly /
R en
ill a
Lu m
in es
ce nc
e
0
0.01
0.02
0.03
0.04
5
10
15
20
*
*
* * * *
25
30
Fig. 4. NMR has higher fidelity of translation than the mouse. Translation fidelity of NMR and mouse skin fibroblasts was quantified as a frequency of amino acid misincorporation into a set of firefly luciferase reporters with a mutated critical lysine 529 (Fig. S3). Misincorporation events can rescue luciferase activity. Luminescence of mutated firefly luciferase was measured and normalized to the luminescence of Renilla luciferase, both under control of the CMV promoter. The positive control (wild-type luciferase) ratio was several orders of magnitude higher and is cropped for visibility. The mutant reporters E – K529E; I – K529I; N – K529N measured misincorporation into the first, second, and third codon positions, respectively; the STOP reporter contained a premature stop codon mutation at amino acid 81; FS+ con- tained a positive frameshift mutation by nucleotide insertion at position 81; and FS contained a negative frameshift mutation by nucleotide deletion at position 81. Results for the assay are shown for NMR and mouse cells grown at 32 °C (A) and 37 °C (B). Error bars show standard deviation. Asterisks in- dicate values significantly different between NMR and mouse (P < 0.005) Student t test.
Azpurua et al. PNAS | October 22, 2013 | vol. 110 | no. 43 | 17353
C EL L B IO LO
G Y
D ow
nl oa
de d
fr om
h tt
ps :/
/w w
w .p
na s.
or g
by 7
3. 20
3. 24
5. 0
on A
pr il
1 2,
2 02
2 fr
om I
P a
dd re
ss 7
3. 20
3. 24
5. 0.
deletion of the major part of the D6 region corresponding to a helix 45 of 23S rRNA in E. coli and leaves two fragments of 28S rRNA disconnected. This may change the folding or dy- namics of the large ribosomal subunit, altering the rate of GTP hydrolysis by elongation factor 1A and/or interaction of the large subunit with tRNA during accommodation, thus affect- ing the fidelity of protein synthesis. In particular, in bacterial ribosome, helix 45 of 23S rRNA is connected to the L11-stalk (L12 stalk in mammals), which is formed by helices 42, 43, and 44 t
Collepals.com Plagiarism Free Papers
Are you looking for custom essay writing service or even dissertation writing services? Just request for our write my paper service, and we'll match you with the best essay writer in your subject! With an exceptional team of professional academic experts in a wide range of subjects, we can guarantee you an unrivaled quality of custom-written papers.
Get ZERO PLAGIARISM, HUMAN WRITTEN ESSAYS
Why Hire Collepals.com writers to do your paper?
Quality- We are experienced and have access to ample research materials.
We write plagiarism Free Content
Confidential- We never share or sell your personal information to third parties.
Support-Chat with us today! We are always waiting to answer all your questions.
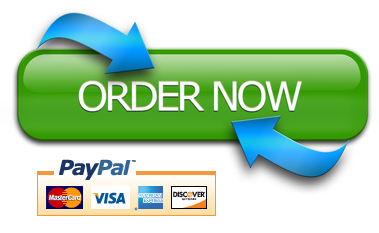