What factors drive how genes are expressed differently in males and females to create the distinct?male?and?female phenotypes? (MO 10.4) b. How can hormone
Discuss the following (total of 150-250 words):
1. Discuss one (1) of the following:
a. What factors drive how genes are expressed differently in males and females to create the distinct male and female phenotypes? (MO 10.4)
b. How can hormones account for sexual orientation? (MO 10.5)
c. Name and describe some of the differences in brain structure that were found between persons who are homosexual and persons who are heterosexual. (MO 10.5)
d. How can a disorder like congenital adrenal hyperplasia explain some differences of sexual orientation in women? (MO 10.5)
e. How is fraternal birth order associated with homosexuality in men? (MO 10.5)
f. For a long time, hormones have been viewed as the main cause behind our behavior. Now, we’re starting to rethink that as we learn more about the body’s complexity. Still, these chemicals clearly have some kind of influence over us. What do you think? Are hormones primarily responsible for our behavior? (MO 10.2)
2. After reading the Stark and Gibbs (2018) article and visiting the InterAct websiteLinks to an external site., choose one (1) of the following interviewsLinks to an external site.: Allie (they/them), Jubi (they/them), Mari (they/them), Bria (they/she), Sophia (she/her). What might be/have been helpful for this person or important others in their lives to have known to improve their experiences living with CAH? (MO 10.6)
CHAPTER 14
Hormones and Development Rachel Stark and Robbin Gibb University of Lethbridge, Lethbridge, AB, Canada
14.1 INTRODUCTION
With the advances in science and technology made in the 20th century, it is remark-
able that it was not until 1959 that a group of researchers began the pioneering studies
in the field of sexual development. Phoenix and colleagues injected pregnant guinea
pigs with testosterone and noted that while the male offspring seemed unaffected, the
prenatal exposure of testosterone created females that were phenotypically male in
characteristic but genetically female (Phoenix, Goy, Gerall, & Young, 1959). Thus,
they published what is known today as the organizational/activational hypothesis of
sexual differentiation. Phoenix and colleagues proposed that the androgens act at a
specific time or times (critical period) during development to permanently alter the
tissue. They suggested a dichotomy between organizational and activational effects.
During the prenatal period the androgens acted to organize the tissue, meaning it pre-
pared certain tissues in the body to respond differently to gonadal hormones in adult-
hood. Then, in adulthood, the gonadal hormones worked to activate the tissues, thus
affecting sexual behavior (Arnold, 2009). Their belief that male hormones changed
the brain was a new and very controversial idea that caused an explosion in the
research of sexual differentiation (Wallen, 2009).
Although this hypothesis has been adapted and changed over time, the main ideas
that Phoenix and his colleagues proposed still hold true today. This chapter will focus
on sexual differentiation, and how this differentiation affects both brain development
and the behaviors that emerge. Factors that impact this development are also dis-
cussed. Finally, we delve into our current understanding of sexual differentiation and
how this idea has changed over time.
14.2 GENETIC FACTORS INFLUENCING SEXUAL DIFFERENTIATION
14.2.1 Sex differences in gene expression Gonadal hormones are the defining factor in sexual differentiation of the body and
brain, but where do these differences in hormones arise? What causes the gonads to
become either testis or ovaries in the first place? This is where genetics plays an
The Neurobiology of Brain and Behavioral Development r 2018 Elsevier Inc. DOI: http://dx.doi.org/10.1016/B978-0-12-804036-2.00014-5 All rights reserved. 391
392 The Neurobiology of Brain and Behavioral Development
important role. Biological sex in humans is determined by the presence or absence of
the Y sex chromosome. During gamete production in males, spermatocytes undergo
division so that the gametes contain half the parents’ genetic material. This results in
sperm that either possesses an X or a Y chromosome. The same process happens dur-
ing female gamete production but since females possess two X chromosomes the
gametes that are formed all contain an X chromosome. This gives rise to the typical
XX female and XY male sex chromosomes once fertilization has occurred (Cheng &
Mruk, 2010).
Gonadal hormones function as secondary factors that act downstream of the pri-
mary factors, the X and Y chromosomes (Arnold, 2009). The expression of genes on
the sex chromosomes influences the sexual differentiation of the brain, and sequen-
tially behavior prior to the onset of gonadal hormone secretion. This suggests that
there is a gene-hormone interaction (Davies & Wilkinson, 2006). The Ar gene encodes the androgen receptor and is found on the X chromosome. This receptor is
critical for male development. Because it is found on the X chromosome, females
carry and express this gene, but circulating progestins, expressed in high levels in
females, inhibit the activity of the receptor. Acting as antiandrogen agents, progestins
block the male typical mode of development (Raudrant & Rabe, 2003). The Ar gene provides a useful example for how genes may encode one mode of development but
the interaction with hormones changes it. It is, therefore, important to understand the
role sex-linked genes have on development (Fig. 14.1). The three mechanisms by
which this may occur include X-linked gene dosage effects, X-linked imprinting, and
Y-specific gene expression.
Figure 14.1 The organization/activation affects the sex chromosomes and hormones have on the brain. Adapted from McCarthy, M.M., & Arnold, A.P. (2011). Reframing sexual differentiation of the brain. Nature Neuroscience, 14(6), 677�683.
Hormones and Development 393
14.2.1.1 X-linked gene dosage Genetic females, as we have established, possess two X chromosomes. This puts
females at risk of potentially fatal levels of gene product from the second X chromo-
some. A mechanism, called x-inactivation, has evolved to cope with this issue. In this
process, one of the X chromosomes is condensed into a Barr body but not all of the
genes on the inactivated chromosome are silenced. In fact, approximately 15% escape
inactivation (Nieschlag, Werler, Wistuba, & Zitzamm, 2014). The escaped genes will
be expressed in higher levels in females than in males, who only have one X chromo-
some. In other words, the genes that escape X-inactivation are more likely to be phe-
notypically expressed in females (Davies & Wilkinson, 2006). For example, color
vision deficiencies like color blindness occur in about 8% of men (according to the
National Eye Institute) while only .5% of the female population is affected. If a female
is missing the functional gene on one chromosome, the other is able to compensate
for this loss. Furthermore, women are able to express tetrachromacy (although this is
fairly rare) as opposed to the normal trichromacy vision due to a double dosing effect
(Jornda, Deeb, Bosten, & Mollon, 2010). This suggests that escaped genes may
account for some of the sexually dimorphic differences seen between males and
females.
14.2.1.2 X-linked imprinting Genomic imprinting involves the preferential expression of a subset of genes that are
marked indicating parental origin. As such, some genes are preferentially expressed
from either the paternal or maternal X chromosome (Reik & Walter, 2001). This
process serves to sexually differentiate males and females as males inherit their X chro-
mosome solely from their mother and females inherit an X chromosome from both
parents. Genetic differences in females arise as the result of expression of the paternal
X chromosome that would not occur in males, while the maternal X chromosome
would be expressed in both sexes (Davis, Isles, Burgoyne, & Wilkinson, 2006). For
example, females with Turner’s Syndrome (possessing only one X chromosome; dis-
cussed in more detail below) show stark differences depending on whether they
received their X chromosome from their mother or father (Iwasa & Pomiankowski,
2001). As such, the parental origin and the preferential expression of genes have been
shown to influence fundamental sex differences in the brain and behavior.
14.2.1.3 Y-specific gene expression The Y chromosome is functionally different from the X chromosome, mainly because
it possesses a region that cannot recombine with the X chromosome during division.
This nonrecombinant region (NRY) has genes on it that are specific to the Y chro-
mosome and comprises 95% of the chromosome’s length. As a result, genes in this
region are only expressed in males (Skaletsky et al., 2003). For example, the Sry gene
394 The Neurobiology of Brain and Behavioral Development
is found on the mammalian Y chromosome and is known as the testis determining
factor (Prokop et al., 2013). This gene is critical for male typical development of the
testis and is another way in which genes account for sexual differentiation.
Originally, it was thought that the Sry gene promoted the growth of the male
reproductive tracts (Wollfian system) and eliminated the female reproductive tracts
(Müllerian system). This in turn caused the production of testosterone, which acts to
masculinize and defeminize the tissues of the body, including the brain. Further
research into the Sry gene has shown that the presence of a Y chromosome may play
a larger role in brain development (McCarthy & Arnold, 2011). In one study,
researchers created phenotypic female mice. The catch is that they had XY chromo-
somes. They did this by removing the Sry gene from the Y chromosome, and as a
result these animals developed in the default female typical pattern (these animals will
be denoted XY2). They furthered this research by mating these genetic XY2 females
and found them to be fertile (Lovell-Badge & Robertson, 1990). More recent studies
using the XY2 females showed that there may be sex differences in the brain due to
the expression of genes found on either the X or Y chromosome. For example, it was
demonstrated that when the Sry gene was present there were more dopaminergic neu-
rons in the brain and further that the XY2 females had significantly lower numbers of
dopaminergic neurons than the XY males. This is thought to be due to the absence
of the Sry gene in the XY2 females (Carruth, Reisert, & Arnold, 2002). Lastly,
research by Dewing et al. (2006) looked at expression of the Sry gene and its protein product. They found that there was increased expression of the Sry gene in the sub- stantia nigra of the midbrain, the thalamus, and, although not to the same extent,
throughout the cortex (Dewing et al., 2006).
14.2.2 Clinical populations One way in which to understand more about the function of the human body, aside
of manipulations to animals (see Chapter 4: The Role of Animal Models in Studying
Brain Development), is to study clinical populations. There are several different sex-
linked gene abnormalities that have been pivotal in understanding the interactions
between genes and hormones, and their effect on sexual differentiation of both the
body and brain.
14.2.2.1 Triple X syndrome Triple X syndrome (XXX) is the addition of an X chromosome, with an incidence
rate of 1/1000 females, that is a result of nondisjunction of the sex chromosomes dur-
ing oogenesis. (Essentially, during gamete development the X chromosomes fail to
separate.) As such, the extra chromosome has a maternal origin (May et al., 1990).
The number of individuals affected may actually be significantly higher as majority of
cases go undiagnosed because some individuals may experience only mild symptoms
Hormones and Development 395
(Gustavson, 1999). Phenotypically, individuals with XXX tend to be tall and thin.
Behaviorally, they show motor coordination difficulties, auditory processing disorders,
psychological and personality disturbances, and an IQ 20 points below the control
level. Anatomically, individuals with XXX have lower total brain volumes and larger
ventricles with reported asymmetries. Some studies report reductions in amygdala
volumes as well (Otter, Schrander-Stumpel, & Crufs, 2009). Most XXX females have
normal ovarian and menstrual functions (Stagi et al., 2016) but occasionally cases of
birth defects (such as overlapping digits) and some of ovarian and menstrual problems
are reported in the literature.
14.2.2.2 Turner syndrome Turner syndrome (TS) is a sex chromosome disorder resulting from complete or par-
tial loss of the X chromosome, affecting 1 in 2000 females. Nondisjunction in gamete
development in either parents results in an individual with only one X chromosome,
XO. This causes a reduction in X-linked gene dosing with the individual only expres-
sing male typical levels of gene expression (Uematsu et al., 2002). Physical characteris-
tics include short stature, infantilism, webbed neck, and cubitus valgus (an abnormal
carrying position for the arm). Along with these physical signs are cognitive deficien-
cies including lowered performance IQ, and impairments in visuospatial skills, short-
term memory, attention, and social interactions (Nijhuis-van der Sanden, Eling, &
Otten, 2003). MRI studies assessing anatomical features of individuals with TS have
found smaller bilateral brain volumes in the hippocampus, caudate, lenticular, thalamic
nuclei (all smaller in the right hemisphere), and the parieto-occipital regions (Murphy
et al., 1993). Moreover, depending on the parental origin of the X chromosome
certain symptoms are exaggerated. For example, individuals that inherited their X
chromosome from their mother lack social awareness, flexibility, and scored lower on
formal tests of social cognitive skills compared to individuals that received their X
chromosome from their father (Skuse et al., 1997).
14.2.2.3 Klinefelters syndrome The most frequent type of congenital chromosomal disorders in males is Klinefelters
syndrome (KS), with a prevalence rate of 1 in 426 to 1 in 1000 (Ngun et al., 2014).
KS is characterized by an extra X chromosome (XXY) and as in females, the second
X chromosome is silenced via X-inactivation and the escaped genes are the cause of
the dosing affects that are seen in KS (Nieschlag, Werler, Wistuba, & Zitzamm, 2014).
Typical symptoms are due to hypogonadism, mainly infertility and testosterone defi-
ciencies as a result of X-linked dosing. Along with these symptoms are those of a
more feminized phenotype including female typical distribution of adipose tissue, and
absent or decreased facial hair. In addition, learning difficulties and a below normal
verbal IQ have been reported (Bojesen, Juul, & Gravholt, 2003). To further
396 The Neurobiology of Brain and Behavioral Development
understand the behavioral deficits observed, researchers have conducted MRI studies
to evaluate the link between brain and behavior. Studies report enlargement of ven-
tricular volume, and a bilateral reduction of cerebellar hemispheres, as well as a signifi-
cant reduction in temporal lobe volume (Itti et al., 2006).
14.2.2.4 Congenital adrenal hyperplasia Congenital adrenal hyperplasia (CAH) occurs when there is a mismatch between
gonadal hormones and sexual differentiation. CAH is the result of an autosomal-
recessive inherited disorder caused by a mutation in the enzyme, 21-hydroxylase,
which is involved in the pathway that converts precursor products into cortisol and
aldosterone. In the case of CAH, these precursors are unable to form the respective
corticosteroids and are metabolized into androgen. This disorder affects both males
and females and occurs in 1 in 15,000 births (White, 2009). Females born with CAH
tend to have ambiguous genitalia due to the high levels of androgens in utero, males
at birth are harder to diagnose without blood tests and tend to go untreated depend-
ing on the severity (Merke & Bornstein, 2005). In the brain, CAH causes a decrease
in amygdalar volume (Merke et al., 2003) and individuals affected with CAH have
symptoms that range from mild to severe depending on the degree of corticosteroid
deficiencies. Behaviorally, there are mixed reviews with some papers reporting
increased IQ and some reporting decreased IQ (Nass & Baker, 1991; Wenzel et al.,
1978). However, females with CAH are reported to have better spatial abilities,
whereas males with CAH are reported to have poorer spatial abilities. This gives some
insight into how the overabundance of androgens may act to organize the tissue of
the developing fetus. Since the discovery in the 1950s that cortisone was an effective
treatment for CAH, the lives of these patients have improved greatly. Screening is
done at birth to detect CAH and treatment can begin (Hampson, Rovet, & Altmann,
1998).
14.2.2.5 Androgen insensitivity Androgen insensitivity (AI) is relatively rare, compared to the other disorders men-
tioned, with a population prevalence of 1 to 5 in 100,000. Individuals with AI are
genetic males, XY. The main pathology of this disorder stems from the lack of func-
tioning androgen receptors. The circulating androgens are at normal levels but the tis-
sues lacking the functioning receptors cannot be activated by androgens and
consequentially develop in a more female typical manner (Cohen-Bendahan, van de
Beek, & Berenbaum, 2005). There are three categories of AI and they have a varying
degree of effects on the body. The first is complete AI syndrome (CAIS), wherein the
tissues of the body are insensitive to androgen (i.e., testosterone) and, consequently,
the external genitalia differentiate in a female-typical direction. Therefore, CAIS indi-
viduals are generally raised as female and are not diagnosed until puberty when they
Hormones and Development 397
fail to menstruate (Ehrhardt & Meyer-Bahlburg, 1979). The second type is mild AI
syndrome (MAIS), this results from the mild impairment of the cells ability to respond
to androgens. The male typical genitalia differentiate during fetal development but
there is impaired development of secondary sexual characteristics during puberty and
infertility (Zuccarello et al., 2008). Partial AI syndrome (PAIS) is the third type.
Partial unresponsiveness to androgens results in impairments in the masculinization of
the male genitalia during fetal development and impairments to male secondary sexual
characteristics. Individuals with PAIS have ambiguous genitalia because not enough
testosterone was available during pregnancy to fully complete the development in a
typical manner (Hughes & Deeb, 2006). Since there is a spectrum in terms of the
etiology of the disorder, there is a varying degree of cognitive sequelae. Impairments in
visuospatial tasks and verbal comprehension have been reported (Imperato-McGlnley,
Plchardo, Gautier, Voyer, & Bryden, 1991).
14.3 ENVIRONMENTAL FACTORS
The relationship the environment has on development has been well established.
Gonadal hormones are an important fetal secondary factor that has the potential to
interact with environmental risk factors and experiences that may impact sexual devel-
opment. Sexual differentiation is vulnerable to pre- and perinatal factors that may
have estrogenic-like or antiandrogenic properties. These deviations can interfere with
development and cause a masculinization or feminization of the brain and as a result,
alter behavior. Here we will consider the effects of prenatal exposures, including
maternal exposure to drugs, nutritional status, and environmental contaminants.
14.3.1 Drugs 14.3.1.1 Nicotine According to the Centers for Disease Control and Prevention (2016), an estimated
15% of US adults report daily smoking. Tobacco use is considered the largest
preventable cause of death and disease. It costs an estimated $96 billion dollars in
direct medical expenses and causes 443,000 smoking related deaths per year (Centers
for Disease Control and Prevention, 2016). Females are particularly susceptible to
developing tobacco-related morbidities and mortalities (Allen, Oncken, & Hatsukami,
2014). Resent research has also suggested that women who smoke have a much lower
success rate of quitting (Piper et al., 2010). Thus, it has been estimated that 10% of
women continue to smoke during pregnancy (Tong et al., 2013).
Alongside a large body of literature indicating the negative impact on overall
health, there is a growing evidence of the consequences of nicotine exposure on the
developing brain. Women who smoke during pregnancy generally have children born
with increased risk for sudden infant death syndrome, low birth weights, attentional
398 The Neurobiology of Brain and Behavioral Development
and cognitive deficits (including learning and memory impairments), and an increased
risk for developing ADHD (Ernst, Moolchan, & Robinson, 2001; Fried, Watkinson,
& Gray, 1998). In rodent studies, prenatal exposure of nicotine reduces birth weight,
increases locomotor activity, and causes poor performance in maze tasks and perma-
nent changes to dendritic morphology (Ernst et al., 2001; Mychasiuk, Muhammad,
Gibb, & Kolb, 2013). These results mimic the deficits observed in the human popula-
tion and provide insight into the etiology of the effects of nicotine.
In the brain, nicotine from tobacco products acts on nicotinic acetylcholine recep-
tors (nAChR), which are ligand-gated channels that mediate the release of the neuro-
transmitter, acetylcholine (Ach). These receptors are expressed in the human brain
during the first trimester. NAChR expression has also been reported to increase dur-
ing critical periods of development, leaving the brain extremely susceptible to envi-
ronmental factors (Dwyer, McQuown, & Leslie, 2009). Sex steroids play a role in the
modulation of the nAChR. Specifically, progesterone and estradiol have been shown
to increase the expression of the genes encoding the nAChR. While progesterone
decreases activity of nAchRs, estradiol increases their activity (Centeno, Henderson,
Pau, Bethea, 2006; Gangitano, Salas, Teng, Perez, & De Biasi, 2009; Jin & Steinbach,
2015; Ke & Lukas, 1996). These findings may shed some light on sex differences
observed with nicotine use in adulthood such as low success for cessation in women,
higher rates of cortisol during withdrawal, and subsequent higher rates of depression
and anxiety during periods of nicotine abstinence (Cross, Linker, & Leslie, 2017).
14.3.1.2 Alcohol Alcohol can readily cross the placenta and as such, it has the potential for affecting
fetal development. Prenatal alcohol exposure can result in fetal alcohol spectrum dis-
order (FASD), with a prevalence rate of 9/1000 births in North America (Thanh &
Jonsson, 2010). FASD presents itself in clinical populations with growth retardation,
impairments in cognition and self-regulation, and substance-use disorders (O’Connor
& Paley, 2009). Prenatal exposure to alcohol has been shown to decrease testosterone
surges, which can lead to feminization of the brain and other tissues in males.
In females, prenatal alcohol exposure has been shown to cause delays in secondary
sexual characteristics later in life, which has been linked to dysregulation of the
hypothalamic�pituitary�adrenal (HPA) axis (see Chapter 16: Socioeconomic Status
for more information). Furthermore, the symptoms seen with prenatal alcohol
exposure may be exacerbated by the changes to the HPA axis in the mother, which
acts as an additional perturbation to the developing fetus (Weinberg, Silwowska, Lan,
& Hellemans, 2008). Alcohol consumption works to increase the activity of the HPA
axis, thereby inducing a stress response in the body. However, chronic alcohol
consumption causes the HPA axis to build up a tolerance to alcohol thereby reducing
Hormones and Development 399
cortisol levels. This tolerance may negatively affect the ability of the HPA axis to
respond to future stressors (Spencer & Hutchison, 1999).
14.3.1.3 Marijuana In 2015, the National Survey on Drug Use and Health found that approximately 20.5
million individuals 18 years of age or older were current users of marijuana, with
117.9 million individuals 12 years of age or older reporting marijuana use at least
once in their lifetime (Center for Behavioral Health Statistics and Quality, 2016).
Although controversy remains on the effects of chronic use on the brain, marijuana is
the most commonly used illegal drug, and its use is on the rise among today’s youth
(Leatherdale, Hammond, & Ahmed, 2008; Wilson et al., 2000).
Individuals who begin smoking marijuana before the age of 17 have brain changes
that include decreases in gray matter and increases white matter. Males who start
smoking early have significantly higher global brain volumes. This has been hypothe-
sized to be a result of the rapid growth observed in the brain during this period of
adolescence (Wilson et al., 2000).
Delta-9-tetrahydrocannabinol (THC) has been implicated in reducing circulating
levels of estradiol and progesterone in females, and testosterone in males. In female
rats, administration of THC has been shown to block ovulation. In rhesus monkeys,
three weekly injections of THC are enough to disrupt normal menstrual cycling; an
effect that lasts for several months. Similar clinical reports have been made in women
who chronically use marijuana. In male rats, THC administration drastically lowers
circulating levels of testosterone (Murphy, Muñoz, Adrian, & Villanúa, 1998).
THC freely crosses the blood brain barrier, the placenta, and is secreted in breast
milk (Kumar et al., 1990). Individuals exposed to marijuana prenatally generally suffer
from poor executive function, working memory, poor attention span, and altered
acoustic profiles of their cries (Eyler & Behnke, 1999; Fried et al., 1998). In rodents,
THC administered to pregnant dams increases reabsorption of pups, and perinatal
exposure has been shown to decrease binding capabilities of dopamine receptors.
Most striking in its effect is the demasculinization of male rats pre- or perinatally
exposed to THC. This could be due to the interaction THC has on GnRH and
therefore decreased circulating testosterone during development (Kumar et al., 1990).
Imaging studies show functional abnormalities in individuals with THC dependence
in the orbitofrontal cortex, insula, basal ganglia, anterior cingul
Collepals.com Plagiarism Free Papers
Are you looking for custom essay writing service or even dissertation writing services? Just request for our write my paper service, and we'll match you with the best essay writer in your subject! With an exceptional team of professional academic experts in a wide range of subjects, we can guarantee you an unrivaled quality of custom-written papers.
Get ZERO PLAGIARISM, HUMAN WRITTEN ESSAYS
Why Hire Collepals.com writers to do your paper?
Quality- We are experienced and have access to ample research materials.
We write plagiarism Free Content
Confidential- We never share or sell your personal information to third parties.
Support-Chat with us today! We are always waiting to answer all your questions.
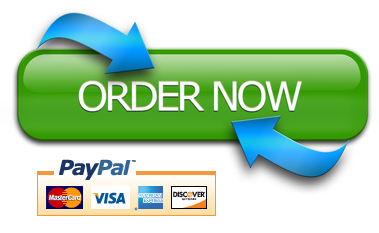