math history problem from Victor J. Katz – A History of Mathematics-Pearson (2008).pdf
A History of MATHEMATICS An Introduction This page intentionally left blank A History of MATHEMATICS An Introduction Third Edition Victor J. Katz University of the District of Columbia Addison-Wesley Boston San Francisco New York London Toronto Sydney Tokyo Singapore Madrid Mexico City Munich Paris Cape Town Hong Kong Montreal To Phyllis, for her patience, encouragement, and love Editor in Chief: Deirdre Lynch Senior Acquisitions Editor: William Hoffman Executive Project Manager: Christine O’Brien Project Editor: Elizabeth Bernardi Associate Editor: Caroline Celano Senior Managing Editor: Karen Wernholm Senior Production Supervisor: Tracy Patruno Marketing Manager: Katie Winter Marketing Assistant: Jon Connelly Senior Prepress Supervisor: Caroline Fell Manufacturing Manager: Evelyn Beaton Production Coordination, Composition, and Illustrations: Windfall Software, using ZzTeX Senior Designer: Barbara T. Atkinson Text and Cover Design: Leslie Haimes Cover photo: Tycho Brahe and Others with Astronomical Instruments, 1587, “Le Quadran Mural” 1663. Blaeu, Joan (1596–1673 Dutch). Newberry Library, Chicago, Illinois, USA © Newberry Library/SuperStock. Many of the designations used by manufacturers and sellers to distinguish their products are claimed as trademarks. Where those designations appear in this book, and Addison-Wesley was aware of a trademark claim, the designations have been printed in initial caps or all caps. Library of Congress Cataloging-in-Publication Data Katz, Victor J. A history of mathematics / Victor Katz.—3rd ed. p. cm. Includes bibliographical references and index. ISBN 0-321-38700-7 1. Mathematics—History. I. Title. QA21.K.33 2009 510.9—dc22 2006049619 Copyright © 2009 by Pearson Education, Inc. All rights reserved. No part of this publication may be reproduced, stored in a retrieval system, or transmitted, in any form or by any means, electronic, mechanical, photocopying, recording, or otherwise, without the prior written permission of the publisher. Printed in the United States of America. For information on obtaining permission for use of material in this work, please submit a written request to Pearson Education, Inc., Rights and Contracts Department, 501 Boylston Street, Suite 900, Boston, MA 02116, fax your request to 617-671-3447, or e-mail at http://www.pearsoned .com/legal/permissions.htm. 1 2 3 4 5 6 7 8 9 10—CRW—12 11 10 09 08 Contents Preface PART ONE . . . . . . . . . . . . . . . . . . . . . . . . . . xi Ancient Mathematics Chapter 1 Egypt and Mesopotamia 1.1 Egypt . . . . . . . . . . . . . . . . . . . . . . . . . 1.2 Mesopotamia . . . . . . . . . . . . . . . . . . . . . . 1.3 Conclusion . . . . . . . . . . . . . . . . . . . . . . . Exercises . . . . . . . . . . . . . . . . . . . . . . . References and Notes . . . . . . . . . . . . . . . . . . 1 2 10 27 28 30 Chapter 2 The Beginnings of Mathematics in Greece 32 2.1 2.2 2.3 The Earliest Greek Mathematics . . . . . . . . . . . . . . The Time of Plato . . . . . . . . . . . . . . . . . . . . Aristotle . . . . . . . . . . . . . . . . . . . . . . . . Exercises . . . . . . . . . . . . . . . . . . . . . . . References and Notes . . . . . . . . . . . . . . . . . . 33 41 43 47 48 Euclid 3.1 Introduction to the Elements . . . . . . . . . . . . . . . . 3.2 Book I and the Pythagorean Theorem . . . . . . . . . . . . 3.3 Book II and Geometric Algebra . . . . . . . . . . . . . . 3.4 Circles and the Pentagon Construction . . . . . . . . . . . . 3.5 Ratio and Proportion . . . . . . . . . . . . . . . . . . . 3.6 Number Theory . . . . . . . . . . . . . . . . . . . . . 3.7 Irrational Magnitudes . . . . . . . . . . . . . . . . . . 3.8 Solid Geometry and the Method of Exhaustion . . . . . . . . 3.9 Euclid’s Data . . . . . . . . . . . . . . . . . . . . . . Exercises . . . . . . . . . . . . . . . . . . . . . . . References and Notes . . . . . . . . . . . . . . . . . . 50 51 53 60 66 71 77 81 83 88 90 92 Chapter 3 vi Contents Chapter 4 Archimedes and Apollonius 4.1 Archimedes and Physics . . . . . . . . . . . . . . . . . 4.2 Archimedes and Numerical Calculations . . . . . . . . . . . 4.3 Archimedes and Geometry . . . . . . . . . . . . . . . . 4.4 Conic Sections before Apollonius . . . . . . . . . . . . . . 4.5 The Conics of Apollonius . . . . . . . . . . . . . . . . . Exercises . . . . . . . . . . . . . . . . . . . . . . . References and Notes . . . . . . . . . . . . . . . . . . 94 96 101 103 112 115 127 131 Chapter 5 Mathematical Methods in Hellenistic Times 133 5.1 5.2 5.3 Astronomy before Ptolemy . . . . . . . . . . . . . . . . Ptolemy and the Almagest . . . . . . . . . . . . . . . . . Practical Mathematics . . . . . . . . . . . . . . . . . . Exercises . . . . . . . . . . . . . . . . . . . . . . . References and Notes . . . . . . . . . . . . . . . . . . 134 145 157 168 170 The Final Chapters of Greek Mathematics 6.1 Nicomachus and Elementary Number Theory . . . . . . . . . 6.2 Diophantus and Greek Algebra . . . . . . . . . . . . . . . 6.3 Pappus and Analysis . . . . . . . . . . . . . . . . . . . 6.4 Hypatia and the End of Greek Mathematics . . . . . . . . . . Exercises . . . . . . . . . . . . . . . . . . . . . . . References and Notes . . . . . . . . . . . . . . . . . . 172 173 176 185 189 191 192 Chapter 6 PART TWO Medieval Mathematics Chapter 7 Ancient and Medieval China 195 7.1 7.2 7.3 7.4 7.5 7.6 196 197 201 209 222 225 226 228 Chapter 8 Introduction to Mathematics in China . . . . . . . . . . . . Calculations . . . . . . . . . . . . . . . . . . . . . . Geometry . . . . . . . . . . . . . . . . . . . . . . . Solving Equations . . . . . . . . . . . . . . . . . . . . Indeterminate Analysis . . . . . . . . . . . . . . . . . . Transmission To and From China . . . . . . . . . . . . . . Exercises . . . . . . . . . . . . . . . . . . . . . . . References and Notes . . . . . . . . . . . . . . . . . . Ancient and Medieval India 230 8.1 8.2 8.3 231 233 237 Introduction to Mathematics in India . . . . . . . . . . . . Calculations . . . . . . . . . . . . . . . . . . . . . . Geometry . . . . . . . . . . . . . . . . . . . . . . . Contents vii Equation Solving . . . . . . . . . . . . . . . . . . . . Indeterminate Analysis . . . . . . . . . . . . . . . . . . Combinatorics . . . . . . . . . . . . . . . . . . . . . Trigonometry . . . . . . . . . . . . . . . . . . . . . . Transmission To and From India . . . . . . . . . . . . . . Exercises . . . . . . . . . . . . . . . . . . . . . . . References and Notes . . . . . . . . . . . . . . . . . . 242 244 250 252 259 260 263 Chapter 9 The Mathematics of Islam 9.1 Introduction to Mathematics in Islam . . . . . . . . . . . . 9.2 Decimal Arithmetic . . . . . . . . . . . . . . . . . . . 9.3 Algebra . . . . . . . . . . . . . . . . . . . . . . . . 9.4 Combinatorics . . . . . . . . . . . . . . . . . . . . . 9.5 Geometry . . . . . . . . . . . . . . . . . . . . . . . 9.6 Trigonometry . . . . . . . . . . . . . . . . . . . . . . 9.7 Transmission of Islamic Mathematics . . . . . . . . . . . . Exercises . . . . . . . . . . . . . . . . . . . . . . . References and Notes . . . . . . . . . . . . . . . . . . 265 266 267 271 292 296 306 317 318 321 Chapter 10 Mathematics in Medieval Europe 10.1 Introduction to the Mathematics of Medieval Europe . . . . . . 10.2 Geometry and Trigonometry . . . . . . . . . . . . . . . . 10.3 Combinatorics . . . . . . . . . . . . . . . . . . . . . 10.4 Medieval Algebra . . . . . . . . . . . . . . . . . . . . 10.5 The Mathematics of Kinematics . . . . . . . . . . . . . . Exercises . . . . . . . . . . . . . . . . . . . . . . . References and Notes . . . . . . . . . . . . . . . . . . 324 325 328 337 342 351 359 362 Chapter 11 Mathematics around the World 11.1 Mathematics at the Turn of the Fourteenth Century . . . . . . . 11.2 Mathematics in America, Africa, and the Pacific . . . . . . . . Exercises . . . . . . . . . . . . . . . . . . . . . . . References and Notes . . . . . . . . . . . . . . . . . . 364 365 370 379 380 8.4 8.5 8.6 8.7 8.8 PART THREE Chapter 12 Early Modern Mathematics Algebra in the Renaissance 383 12.1 The Italian Abacists . . . . . . . . . . . . . . . . . . . 12.2 Algebra in France, Germany, England, and Portugal . . . . . . 12.3 The Solution of the Cubic Equation . . . . . . . . . . . . . 385 389 399 viii Contents 12.4 Viète, Algebraic Symbolism, and Analysis . . . . . . . . . . 12.5 Simon Stevin and Decimal Fractions . . . . . . . . . . . . Exercises . . . . . . . . . . . . . . . . . . . . . . . References . . . . . . . . . . . . . . . . . . . . . . 407 414 418 420 Chapter 13 Mathematical Methods in the Renaissance 13.1 Perspective . . . . . . . . . . . . . . . . . . . . . . . 13.2 Navigation and Geography . . . . . . . . . . . . . . . . 13.3 Astronomy and Trigonometry . . . . . . . . . . . . . . . 13.4 Logarithms . . . . . . . . . . . . . . . . . . . . . . 13.5 Kinematics . . . . . . . . . . . . . . . . . . . . . . . Exercises . . . . . . . . . . . . . . . . . . . . . . . References and Notes . . . . . . . . . . . . . . . . . . 423 427 432 435 453 457 462 464 Chapter 14 Algebra, Geometry, and Probability in the Seventeenth Century 14.1 The Theory of Equations . . . . . . . . . . . . . . . . . 14.2 Analytic Geometry . . . . . . . . . . . . . . . . . . . 14.3 Elementary Probability . . . . . . . . . . . . . . . . . . 14.4 Number Theory . . . . . . . . . . . . . . . . . . . . . 14.5 Projective Geometry . . . . . . . . . . . . . . . . . . . Exercises . . . . . . . . . . . . . . . . . . . . . . . References and Notes . . . . . . . . . . . . . . . . . . 467 468 473 487 497 499 501 504 Chapter 15 The Beginnings of Calculus 15.1 Tangents and Extrema . . . . . . . . . . . . . . . . . . 15.2 Areas and Volumes . . . . . . . . . . . . . . . . . . . 15.3 Rectification of Curves and the Fundamental Theorem . . . . . Exercises . . . . . . . . . . . . . . . . . . . . . . . References and Notes . . . . . . . . . . . . . . . . . . 507 509 514 532 539 541 Chapter 16 Newton and Leibniz 16.1 Isaac Newton . . . . . . . . . . . . . . . . . . . . . . 16.2 Gottfried Wilhelm Leibniz . . . . . . . . . . . . . . . . 16.3 First Calculus Texts . . . . . . . . . . . . . . . . . . . Exercises . . . . . . . . . . . . . . . . . . . . . . . References and Notes . . . . . . . . . . . . . . . . . . 543 544 565 575 579 580 PART FOUR Chapter 17 Modern Mathematics Analysis in the Eighteenth Century 583 17.1 Differential Equations . . . . . . . . . . . . . . . . . . 17.2 The Calculus of Several Variables . . . . . . . . . . . . . . 584 601 Contents ix 17.3 Calculus Texts . . . . . . . . . . . . . . . . . . . . . 17.4 The Foundations of Calculus . . . . . . . . . . . . . . . . Exercises . . . . . . . . . . . . . . . . . . . . . . . References and Notes . . . . . . . . . . . . . . . . . . 611 628 636 639 Chapter 18 Probability and Statistics in the Eighteenth Century 18.1 Theoretical Probability . . . . . . . . . . . . . . . . . . 18.2 Statistical Inference . . . . . . . . . . . . . . . . . . . 18.3 Applications of Probability . . . . . . . . . . . . . . . . Exercises . . . . . . . . . . . . . . . . . . . . . . . References and Notes . . . . . . . . . . . . . . . . . . 642 643 651 655 661 663 Chapter 19 Algebra and Number Theory in the Eighteenth Century 19.1 Algebra Texts . . . . . . . . . . . . . . . . . . . . . 19.2 Advances in the Theory of Equations . . . . . . . . . . . . 19.3 Number Theory . . . . . . . . . . . . . . . . . . . . . 19.4 Mathematics in the Americas . . . . . . . . . . . . . . . Exercises . . . . . . . . . . . . . . . . . . . . . . . References and Notes . . . . . . . . . . . . . . . . . . 665 666 671 677 680 683 684 Chapter 20 Geometry in the Eighteenth Century 20.1 Clairaut and the Elements of Geometry . . . . . . . . . . . . 20.2 The Parallel Postulate . . . . . . . . . . . . . . . . . . 20.3 Analytic and Differential Geometry . . . . . . . . . . . . . 20.4 The Beginnings of Topology . . . . . . . . . . . . . . . . 20.5 The French Revolution and Mathematics Education . . . . . . Exercises . . . . . . . . . . . . . . . . . . . . . . . References and Notes . . . . . . . . . . . . . . . . . . 686 687 689 695 701 702 706 707 Chapter 21 Algebra and Number Theory in the Nineteenth Century 21.1 Number Theory . . . . . . . . . . . . . . . . . . . . . 21.2 Solving Algebraic Equations . . . . . . . . . . . . . . . . 21.3 Symbolic Algebra . . . . . . . . . . . . . . . . . . . . 21.4 Matrices and Systems of Linear Equations . . . . . . . . . . 21.5 Groups and Fields—The Beginning of Structure . . . . . . . . Exercises . . . . . . . . . . . . . . . . . . . . . . . References and Notes . . . . . . . . . . . . . . . . . . 709 711 721 730 740 750 759 761 Chapter 22 Analysis in the Nineteenth Century 764 22.1 Rigor in Analysis . . . . . . . . . . . . . . . . . . . . 22.2 The Arithmetization of Analysis . . . . . . . . . . . . . . 22.3 Complex Analysis . . . . . . . . . . . . . . . . . . . . 766 788 795 x Contents 22.4 Vector Analysis . . . . . . . . . . . . . . . . . . . . . Exercises . . . . . . . . . . . . . . . . . . . . . . . References and Notes . . . . . . . . . . . . . . . . . . 807 813 815 Chapter 23 Probability and Statistics in the Nineteenth Century 23.1 The Method of Least Squares and Probability Distributions . . . 23.2 Statistics and the Social Sciences . . . . . . . . . . . . . . 23.3 Statistical Graphs . . . . . . . . . . . . . . . . . . . . Exercises . . . . . . . . . . . . . . . . . . . . . . . References and Notes . . . . . . . . . . . . . . . . . . 818 819 824 828 831 831 Chapter 24 Geometry in the Nineteenth Century 24.1 Differential Geometry . . . . . . . . . . . . . . . . . . 24.2 Non-Euclidean Geometry . . . . . . . . . . . . . . . . . 24.3 Projective Geometry . . . . . . . . . . . . . . . . . . . 24.4 Graph Theory and the Four-Color Problem . . . . . . . . . . 24.5 Geometry in N Dimensions . . . . . . . . . . . . . . . . 24.6 The Foundations of Geometry . . . . . . . . . . . . . . . Exercises . . . . . . . . . . . . . . . . . . . . . . . References and Notes . . . . . . . . . . . . . . . . . . 833 835 839 852 858 862 867 870 872 Chapter 25 Aspects of the Twentieth Century and Beyond 25.1 Set Theory: Problems and Paradoxes . . . . . . . . . . . . 25.2 Topology . . . . . . . . . . . . . . . . . . . . . . . 25.3 New Ideas in Algebra . . . . . . . . . . . . . . . . . . 25.4 The Statistical Revolution . . . . . . . . . . . . . . . . . 25.5 Computers and Applications . . . . . . . . . . . . . . . . 25.6 Old Questions Answered . . . . . . . . . . . . . . . . . Exercises . . . . . . . . . . . . . . . . . . . . . . . References and Notes . . . . . . . . . . . . . . . . . . 874 876 882 890 903 907 919 926 928 Appendix A Using This Textbook in Teaching Mathematics A.1 Courses and Topics . . . . . . . . . . . . . . . . . . . A.2 Sample Lesson Ideas to Incorporate History . . . . . . . . . . A.3 Time Line . . . . . . . . . . . . . . . . . . . . . . . 931 931 935 939 General References in the History of Mathematics . . . . . . . . . 945 Answers to Selected Exercises . . . . . . . . . . . . . . . . . 949 Index and Pronunciation Guide . . . . . . . . . . . . . . . . . 961 Preface In A Call For Change: Recommendations for the Mathematical Preparation of Teachers of Mathematics, the Mathematical Association of America’s (MAA) Committee on the Mathematical Education of Teachers recommends that all prospective teachers of mathematics in schools . . . develop an appreciation of the contributions made by various cultures to the growth and development of mathematical ideas; investigate the contributions made by individuals, both female and male, and from a variety of cultures, in the development of ancient, modern, and current mathematical topics; [and] gain an understanding of the historical development of major school mathematics concepts. According to the MAA, knowledge of the history of mathematics shows students that mathematics is an important human endeavor. Mathematics was not discovered in the polished form of our textbooks, but was often developed in an intuitive and experimental fashion in order to solve problems. The actual development of mathematical ideas can be effectively used in exciting and motivating students today. This textbook grew out of the conviction that both prospective school teachers of mathematics and prospective college teachers of mathematics need a background in history to teach the subject more effectively. It is therefore designed for junior or senior mathematics majors who intend to teach in college or high school, and it concentrates on the history of those topics typically covered in an undergraduate curriculum or in elementary or high school. Because the history of any given mathematical topic often provides excellent ideas for teaching the topic, there is sufficient detail in each explanation of a new concept for the future (or present) teacher of mathematics to develop a classroom lesson or series of lessons based on history. In fact, many of the problems ask readers to develop a particular lesson. My hope is that students and prospective teachers will gain from this book a knowledge of how we got here from there, a knowledge that will provide a deeper understanding of many of the important concepts of mathematics. Distinguishing Features FLEXIBLE ORGANIZATION Although the text’s chief organization is by chronological period, the material is organized topically within each period. By consulting the detailed subsection headings, the reader can choose to follow a particular theme throughout history. For example, to study equation solving one could consider ancient Egyptian and Babylonian methods, the geometrical solution methods of the Greeks, the numerical methods of the Chinese, the Islamic solution methods for cubic equations by use of conic sections, the Italian discovery of an algorithmic solution of cubic and quartic equations, the work of Lagrange in developing criteria for methods of xii Preface solution of higher degree polynomial equations, the work of Gauss in solving cyclotomic equations, and the work of Galois in using permutations to formulate what is today called Galois theory. FOCUS ON TEXTBOOKS It is one thing to do mathematical research and discover new theorems and techniques. It is quite another to elucidate these in such a way that others can learn them. Thus, in many chapters there is a discussion of one or more important texts of the time. These are the works from which students learned the important ideas of the great mathematicians. Today’s students will see how certain topics were treated and will be able to compare these treatments to those in current texts and see the kinds of problems students of years ago were expected to solve. APPLICATIONS OF MATHEMATICS Two chapters, one for the Greek period and one for the Renaissance, are devoted entirely to mathematical methods, the ways in which mathematics was used to solve problems in other areas of study. A major part of both chapters deals with astronomy since in ancient times astronomers and mathematicians were usually the same people. To understand a substantial part of Greek mathematics, it is crucial also to understand the Greek model of the heavens and how mathematics was used in applying this model to give predictions. Similarly, I discuss the Copernicus-Kepler model of the heavens and consider how mathematicians of the Renaissance applied mathematics to its study. I also look at the applications of mathematics to geography during these two time periods. NON-WESTERN MATHEMATICS A special effort has been made to consider mathematics developed in parts of the world other than Europe. Thus, there is substantial material on mathematics in China, India, and the Islamic world. In addition, Chapter 11 discusses the mathematics of various other societies around the world. Readers will see how certain mathematical ideas have occurred in many places, although not perhaps in the context of what we in the West call “mathematics.” TOPICAL EXERCISES Each chapter contains many exercises, organized in order of the chapter’s topics. Some exercises are simple computational ones, while others help to fill gaps in the mathematical arguments presented in the text. For Discussion exercises are open-ended questions, which may involve some research to find answers. Many of these ask students to think about how they would use historical material in the classroom. Even if readers do not attempt many of the exercises, they should at least read them to gain a fuller understanding of the material of the chapter. (Answers to the odd numbered computational problems as well as some odd numbered “proof” problems are included at the end of the book.) FOCUS ESSAYS Biographies For easy reference, many biographies of the mathematicians whose work is discussed are in separate boxes. Although women have for various reasons not participated in large numbers in mathematical research, biographies of several important women mathematicians are included, women who succeeded, usually against heavy odds, in contributing to the mathematical enterprise. Preface xiii Special Topics Sidebars on special topics also appear throughout the book. These include such items as a treatment of the question of the Egyptian influence on Greek mathematics, a discussion of the idea of a function in the work of Ptolemy, a comparison of various notions of continuity, and several containing important definitions collected together for easy reference. ADDITIONAL PEDAGOGY At the start of each chapter is a relevant quotation and a description of an important mathematical “event.” Each chapter also contains an annotated list of references to both primary and secondary sources from which students can obtain more information. Given that a major audience for this text is prospective teachers of secondary or college-level mathematics, I have provided an appendix giving suggestions for using the text material in teaching mathematics. It contains a detailed list to correlate the history of various topics in the secondary and college curriculum to sections in the text; there are suggestions for organizing some of this material for classroom use; and there is a detailed time line that helps to relate the mathematical discoveries to other events happening in the world. On the back inside cover there is a chronological listing of most of the mathematicians discussed in the book. Finally, given that students may have difficulty pronouncing the names of some mathematicians, the index has a special feature: a phonetic pronunciation guide. Prerequisites A knowledge of calculus is sufficient to understand the first 16 chapters of the text. The mathematical prerequisites for later chapters are somewhat more demanding, but the various section titles indicate clearly what kind of mathematical knowledge is required. For example, a full understanding of chapters 19 and 21 will require that students have studied abstract algebra. Course Flexibility The text contains more material than can be included in a typical one-semester course in the history of mathematics. In fact, it includes adequate material for a full year course, the first half being devoted to the period through the invention of calculus in the late seventeenth century and the second half covering the mathematics of the eighteenth, nineteenth, and twentieth centuries. However, for those instructors who have only one semester, there are several ways to use this book. First, one could cover most of the first twelve chapters and simply conclude with calculus. Second, one could choose to follow one or two particular themes through history. (The table in the appendix will direct one to the appropriate sections to include when dealing with a particular theme.) Among the themes that could be followed are equation solving; ideas of calculus; concepts of geometry; trigonometry and its applications to astronomy and surveying; combinatorics, probability, and statistics; and modern algebra and number theory. For a thematic approach, I would suggest making every effort to include material on mathematics in the twentieth century, to help students realize that new mathematics is continually being discovered. Finally, one could combine the two approaches and cover ancient times chronologically, and then pick a theme for the modern era. xiv Preface New for this Edition The generally friendly reception of this text’s first two editions encouraged me to maintain the basic organization and content. Nevertheless, I have attempted to make a number of improvements, both in clarity and in content, based on comments from many users of those editions as well as new discoveries in the history of mathematics that have appeared in the recent literature. To make the book somewhat easier to use, I have reorganized some material into shorter chapters. There are minor changes in virtually every section, but the major changes from the second edition include: new material about Archimedes discovered in analyzing the palimpsest of the Method; a new section on Ptolemy’s Geography; more material in the Chinese, Indian, and Islamic chapters based on my work on the new Sourcebook dealing with the mathematics of these civilizations, as well as the ancient Egyptian and Babylonian ones; new material on statistics in the nineteenth and twentieth centuries; and a description of the eighteenth-century translation into the differential calculus of some of Newton’s work in the Principia. The text concludes with a brief description of the solution to the first Clay Institute problem, the Poincarè conjecture. I have attempted to correct all factual errors from the earlier editions without introducing new ones, yet would appreciate notes from anyone who discovers any remaining errors. New problems appear in every chapter, some of them easier ones, and references to the literature have been updated wherever possible. Also, a few new stamps were added as illustrations. One should note, however, that any portraits on these stamps—or indeed elsewhere—purporting to represent mathematicians before the sixteenth century are fictitious. There are no known representations of any of these people that have credible evidence of being authentic. Acknowledgments Like any book, this one could not have been written without the help of many people. The following people have read large sections of the book at my request and have offered many valuable suggestions: Marcia Ascher (Ithaca College); J. Lennart Berggren (Simon Fraser University); Robert Kreiser (A.A.U.P.); Robert Rosenfeld (Nassau Community College); John Milcetich (University of the District of Columbia); Eleanor Robson (Cambridge University); and Kim Plofker (Brown University). In addition, many people made detailed suggestions for the second and third editions. Although I have not followed every suggestion, I sincerely appreciate the thought they gave toward improving the book. These people include Ivor Grattan-Guinness, Richard Askey, William Anglin, Claudia Zaslavsky, Rebekka Struik, William Ramaley, Joseph Albree, Calvin Jongsma, David Fowler, John Stillwell, Christian Thybo, Jim Tattersall, Judith Grabiner, Tony Gardiner, Ubi D’Ambrosio, Dirk Struik, and David Rowe. My heartfelt thanks to all of them. The many reviewers of sections of the manuscript for each of the editions have also provided great help with their detailed critiques and have made this a much better book than it otherwise could have been. For the first edition, they were Duane Blumberg (University of Southwestern Louisiana); Walter Czarnec (Framington State University); Joseph Dauben (Lehman College–CUNY); Harvey Davis (Michigan State University); Joy Easton (West Virginia University); Carl FitzGerald (University of California, San Diego); Basil Gordon (University of California, Los Angeles); Mary Gray (American University); Branko Grun- Preface xv baum (University of Washington); William Hintzman (San Diego State University); Barnabas Hughes (California State University, Northridge); Israel Kleiner (York University); David E. Kullman (Miami University); Robert L. Hall (University of Wisconsin, Milwaukee); Richard Marshall (Eastern Michigan University); Jerold Mathews (Iowa State University); Willard Parker (Kansas State University); Clinton M. Petty (University of Missouri, Columbia); Howard Prouse (Mankato State University); Helmut Rohrl (University of California, San Diego); David Wilson (University of Florida); and Frederick Wright (University of North Carolina at Chapel Hill). For the second edition, the reviewers were Salvatore Anastasio (State University of New York, New Paltz); Bruce Crauder (Oklahoma State University); Walter Czarnec (Framingham State College); William England (Mississippi State University); David Jabon (Eastern Washington University); Charles Jones (Ball State University); Michael Lacey (Indiana University); Harold Martin (Northern Michigan University); James Murdock (Iowa State University); Ken Shaw (Florida State University); Svere Smalo (University of California, Santa Barbara); Domina Eberle Spencer (University of Connecticut); and Jimmy Woods (North Georgia College). For the third edition, the reviewers were Edward Boamah (Blackburn College); Douglas Cashing (St. Bonaventure University); Morley Davidson (Kent State University); Martin J. Erickson (Truman State University); Jian-Guo Liu (University of Maryland); Warren William McGovern (Bowling Green State University); Daniel E. Otero (Xavier University); Talmage James Reid (University of Mississippi); Angelo Segalla (California State University, Long Beach); Lawrence Shirley (Towson University); Agnes Tuska (California State University at Fresno); Jeffrey X. Watt (Indiana University–Purdue University Indianapolis). I have also benefited greatly from conversations with many historians of mathematics at various forums, including numerous history sessions at the annual joint meetings of the Mathematical Association of America and the American Mathematical Society. Among those who have helped at various stages (and who have not been mentioned earlier) are V. Frederick Rickey, United States Military Academy; Florence Fasanelli, AAAS; Israel Kleiner, York University; Abe Shenitzer, York University; Frank Swetz, Pennsylvania State University, and Janet Beery, University of Redlands. In addition, I want to thank Karen Dee Michalowicz, of the Langley School, who showed me how to reach current and prospective high school teachers, and whose untimely death in 2006 was such a tragedy. In addition, I learned a lot from all the people who attended various sessions of the Institute in the History of Mathematics and Its Use in Teaching, as well as members of the 2007 PREP workshop on Asian mathematics. My students in History of Mathematics classes (and others) at the University of the District of Columbia have also helped me clarify many of my ideas. Naturally, I welcome any additional comments and correspondence from students and colleagues elsewhere in an effort to continue to improve this book. My former editors at Harper Collins, Steve Quigley, Don Gecewicz, and George Duda, who helped form the first edition, and Jennifer Albanese, who was the editor for the second edition, were very helpful. And I want to particularly thank my new editor, Bill Hoffman, for all his suggestions and his support during the creation of both the brief edition and this new third edition. Elizabeth Bernardi at Pearson Addison-Wesley has worked hard to keep me on deadline, and Jean-Marie Magnier has caught several errors in the answers to problems, xvi Preface for which I thank her. The production staff of Paul C. Anagnostopoulos, Jennifer McClain, Laurel Muller, Yonie Overton, and Joe Snowden have cheerfully and efficiently handled their tasks to make this book a reality. Lastly, I want to thank my wife Phyllis for all her love and support over the years, during the very many hours of working on this book and, of course, during the other hours as well. Victor J. Katz Silver Spring, MD May 2008 1 PART ONE Ancient Mathematics chapter Egypt and Mesopotamia Accurate reckoning. The entrance into the knowledge of all existing things and all obscure secrets. —Introduction to Rhind Mathematical Papyrus1 M esopotamia: In a scribal school in Larsa some 3800 years ago, a teacher is trying to develop mathematics problems to assign to his students so they can practice the ideas just introduced on the relationship among the sides of a right triangle. The teacher not only wants the computations to be difficult enough to show him who really understands the material but also wants the answers to come out as whole numbers so the students will not be frustrated. After playing for several hours with the few triples (a, b, c) of numbers he knows that satisfy a 2 + b2 = c2, a new idea occurs to him. With a few deft strokes of his stylus, he quickly does some calculations on a moist clay tablet and convinces himself that he has discovered how to generate as many of these triples as necessary. After organizing his thoughts a bit longer, he takes a fresh tablet and carefully records a table listing not only 15 such triples but also a brief indication of some of the preliminary calculations. He does not, however, record the details of his new method. Those will be saved for his lecture to his colleagues. They will then be forced to acknowledge his abilities, and his reputation as one of the best teachers of mathematics will spread throughout the entire kingdom. 1 2 Chapter 1 Egypt and Mesopotamia The opening quotation from one of the few documentary sources on Egyptian mathematics and the fictional story of the Mesopotamian scribe illustrate some of the difficulties in giving an accurate picture of ancient mathematics. Mathematics certainly existed in virtually every ancient civilization of which there are records. But in every one of these civilizations, mathematics was in the domain of specially trained priests and scribes, government officials whose job it was to develop and use mathematics for the benefit of that government in such areas as tax collection, measurement, building, trade, calendar making, and ritual practices. Yet, even though the origins of many mathematical concepts stem from their usefulness in these contexts, mathematicians always exercised their curiosity by extending these ideas far beyond the limits of practical necessity. Nevertheless, because mathematics was a tool of power, its methods were passed on only to the privileged few, often through an oral tradition. Hence, the written records are generally sparse and seldom provide much detail. In recent years, however, a great deal of scholarly effort has gone into reconstructing the mathematics of ancient civilizations from whatever clues can be found. Naturally, all scholars do not agree on every point, but there is enough agreement so that a reasonable picture can be presented of the mathematical knowledge of the ancient civilizations in Mesopotamia and Egypt. We begin our discussion of the mathematics of each of these civilizations with a brief survey of the underlying civilization and a description of the sources from which our knowledge of the mathematics is derived. 1.1 FIGURE 1.1 Jean Champollion and a piece of the Rosetta stone EGYPT Agriculture emerged in the Nile Valley in Egypt close to 7000 years ago, but the first dynasty to rule both Upper Egypt (the river valley) and Lower Egypt (the delta) dates from about 3100 bce. The legacy of the first pharaohs included an elite of officials and priests, a luxurious court, and for the kings themselves, a role as intermediary between mortals and gods. This role fostered the development of Egypt’s monumental architecture, including the pyramids, built as royal tombs, and the great temples at Luxor and Karnak. Writing began in Egypt at about this time, and much of the earliest writing concerned accounting, primarily of various types of goods. There were several different systems of measuring, depending on the particular goods being measured. But since there were only a limited number of signs, the same signs meant different things in connection with different measuring systems. From the beginning of Egyptian writing, there were two styles, the hieroglyphic writing for monumental inscriptions and the hieratic, or cursive, writing, done with a brush and ink on papyrus. Greek domination of Egypt in the centuries surrounding the beginning of our era was responsible for the disappearance of both of these native Egyptian writing forms. Fortunately, Jean Champollion (1790–1832) was able to begin the process of understanding Egyptian writing early in the nineteenth century through the help of a multilingual inscription—the Rosetta stone—in hieroglyphics and Greek as well as the later demotic writing, a form of the hieratic writing of the papyri (Fig. 1.1). It was the scribes who fostered the development of the mathematical techniques. These government officials were crucial to ensuring the collection and distribution of goods, thus helping to provide the material basis for the pharaohs’ rule (Fig. 1.2). Thus, evidence for the techniques comes from the education and daily work of the scribes, particularly as related in 1.1 Egypt 3 two papyri containing collections of mathematical problems with their solutions, the Rhind Mathematical Papyrus, named for the Scotsman A. H. Rhind (1833–1863) who purchased it at Luxor in 1858, and the Moscow Mathematical Papyrus, purchased in 1893 by V. S. Golenishchev (d. 1947) who later sold it to the Moscow Museum of Fine Arts. The former papyrus was copied about 1650 bce by the scribe A’h-mose from an original about 200 years older and is approximately 18 feet long and 13 inches high. The latter papyrus dates from roughly the same period and is over 15 feet long, but only some 3 inches high. Unfortunately, although a good many papyri have survived the ages due to the generally dry Egyptian climate, it is the case that papyrus is very fragile. Thus, besides the two papyri mentioned, only a few short fragments of other original Egyptian mathematical papyri are still extant. FIGURE 1.2 Amenhotep, an Egyptian high official and scribe (fifteenth century bce) These two mathematical texts inform us first of all about the types of problems that needed to be solved. The majority of problems were concerned with topics involving the administration of the state. That scribes were occupied with such tasks is shown by illustrations found on the walls of private tombs. Very often, in tombs of high officials, scribes are depicted working together, probably in accounting for cattle or produce. Similarly, there exist threedimensional models representing such scenes as the filling of granaries, and these scenes always include a scribe to record quantities. Thus, it is clear that Egyptian mathematics was developed and practiced in this practical context. One other area in which mathematics played an important role was architecture. Numerous remains of buildings demonstrate that mathematical techniques were used both in their design and construction. Unfortunately, there are few detailed accounts of exactly how the mathematics was used in building, so we can only speculate about many of the details. We deal with a few of these ideas below. 1.1.1 Number Systems and Computations The Egyptians developed two different number systems, one for each of their two writing styles. In the hieroglyphic system, each of the first several powers of 10 was represented by a different symbol, beginning with the familiar vertical stroke for 1. Thus, 10 was represented by ∩, 100 by , 1000 by , and 10,000 by (Fig. 1.3). Arbitrary whole numbers were then represented by appropriate repetitions of the symbols. For example, to represent 12,643 the Egyptians would write . (Note that the usual practice was to put the smaller digits on the left.) FIGURE 1.3 Egyptian numerals on the Naqada tablets (c. 3000 bce) 4 Chapter 1 Egypt and Mesopotamia The hieratic system, in contrast to the hieroglyphic, is an example of a ciphered system. Here each number from 1 to 9 had a specific symbol, as did each multiple of 10 from 10 to 90 and each multiple of 100 from 100 to 900, and so on. A given number, for example, 37, was written by putting the symbol for 7 next to that for 30. Since the symbol for 7 was and that for 30 was , 37 was written . Again, since 3 was written as , 40 as , and 200 as , the symbol for 243 was . Although a zero symbol is not necessary in a ciphered system, the Egyptians did have such a symbol. This symbol does not occur in the mathematical papyri, however, but in papyri dealing with architecture, where it is used to denote the bottom leveling line in the construction of a pyramid, and accounting, where it is used in balance sheets to indicate that the disbursements and income are equal.2 Once there is a system of writing numbers, it is only natural that a civilization devise algorithms for computation with these numbers. For example, in Egyptian hieroglyphics, addition and subtraction are quite simple: combine the units, then the tens, then the hundreds, and so on. Whenever a group of ten of one type of symbol appears, replace it by one of the next. Hence, to add 783 and 275, put and together to get . Since there are fifteen ∩’s, replace ten of them by one . This then gives ten of the latter. Replace these by one . The final answer is , or 1058. Subtraction is done similarly. Whenever “borrowing” is needed, one of the symbols would be converted to ten of the next lower symbol. Such a simple algorithm for addition and subtraction is not possible in the hieratic system. Probably, the scribes simply memorized basic addition tables. The Egyptian algorithm for multiplication was based on a continual doubling process. To multiply two numbers a and b, the scribe would first write down the pair 1, b. He would then double each number in the pair repeatedly, until the next doubling would cause the first element of the pair to exceed a. Then, having determined the powers of 2 that add to a, the scribe would add the corresponding multiples of b to get his answer. For example, to multiply 12 by 13, the scribe would set down the following lines: 1 2 4 8 12 24 48 96 At this point he would stop because the next doubling would give him 16 in the first column, which is larger than 13. He would then check off those multipliers that added to 13, namely, 1, 4, and 8, and add the corresponding numbers in the other column. The result would be written as follows: Totals 13 156. There is no record of how the scribe did the doubling. The answers are simply written down. Perhaps the scribe had memorized an extensive two times table. In fact, there is some evidence that doubling was a standard method of computation in areas of Africa to the south of Egypt 1.1 Egypt 5 and that therefore the Egyptian scribes learned from their southern colleagues. In addition, the scribes were somehow aware that every positive integer could be uniquely expressed as the sum of powers of two. That fact provides the justification for the procedure. How was it discovered? The best guess is that it was discovered by experimentation and then passed down as tradition. Because division is the inverse of multiplication, a problem such as 156 ÷ 12 would be stated as, “multiply 12 so as to get 156.” The scribe would then write down the same lines as above. This time, however, he would check off the lines having the numbers in the right-hand column that sum to 156; here that would be 12, 48, and 96. Then the sum of the corresponding numbers on the left, namely, 1, 4, and 8, would give the answer 13. Of course, division does not always “come out even.” When it did not, the Egyptians resorted to fractions. The Egyptians only dealt with unit fractions or “parts” (fractions with numerator 1), with the single exception of 2/3, perhaps because these fractions are the most “natural.” The fraction 1/n (the nth part) is in general represented in hieroglyphics by the symbol for the integer n with the symbol above. In the hieratic a dot is used instead. So 1/7 is denoted in the former system by and in the latter by . The single exception, 2/3, had a special symbol: in hieroglyphic and in hieratic. Two other fractions, 1/2 and 1/4, also had special symbols: and x, respectively. In what follows, however, the notation n will be used to represent 1/n and 3 to represent 2/3. Because fractions show up as the result of divisions that do not come out evenly, surely there is a need to be able to deal with fractions other than unit fractions. It was in this connection that the most intricate of the Egyptian arithmetical techniques developed, the representation of any fraction in terms of unit fractions. The Egyptians did not view the question this way, however. Whenever we would use a nonunit fraction, they simply wrote a sum of unit fractions. For example, problem 3 of the Rhind Mathematical Papyrus asks how to divide 6 loaves among 10 men. The answer is given that each man gets 2 10 loaves (that is, 1/2 + 1/10). The scribe checks this by multiplying this value by 10. We may regard the scribe’s answer as more cumbersome than our answer of 3/5, but in some sense the actual division is easier to accomplish this way. We divide five of the loaves in half, the sixth one in tenths, and then give each man one half plus one tenth. It is then clear to all that every man has the same portion of bread. Cumbersome or not, this Egyptian method of unit fractions was used throughout the Mediterranean basin for over 2000 years. In multiplying whole numbers, the important step is the doubling step. So too in multiplying fractions; the scribe had to be able to express the double of any unit fraction. For example, in the problem above, the check of the solution is written as follows: 1 2 10 2 15 4 2 3 15 8 4 3 10 30 10 6 How are these doubles formed? To double 2 10 is easy; because each denominator is even, each is merely halved. In the next line, however, 5 must be doubled. It was here that the 6 Chapter 1 Egypt and Mesopotamia scribe had to use a table to get the answer 3 15 (that is, 2 . 1/5 = 1/3 + 1/15). In fact, the first section of the Rhind Papyrus is a table of the division of 2 by every odd integer from 3 to 101 (Fig. 1.4), and the Egyptian scribes realized that the result of multiplying n by 2 is the same as that of dividing 2 by n. It is not known how the division table was constructed, but there are several scholarly accounts giving hypotheses for the scribes’ methods. In any case, the solution of problem 3 depends on using that table twice, first as already indicated and second in the next step, where the double of 15 is given as 10 30 (or 2 . 1/15 = 1/10 + 1/30). The final step in this problem involves the addition of 1 5 to 4 3 10 30, and here the scribe just gave the answer. Again, the conjecture is that for such addition problems an extensive table existed. The Egyptian Mathematical Leather Roll, which dates from about 1600 bce, contains a short version of such an addition table.3 There are also extant several other tables for dealing with unit fractions and a multiplication table for the special fraction 2/3. It thus appears that the arithmetic algorithms used by the Egyptian scribes involved extensive knowledge of FIGURE 1.4 Transcription and hieroglyphic translation of 2 ÷ 3, 2 ÷ 5, and 2 ÷ 7 from the Rhind Mathematical Papyrus (Reston, VA: National Council of Teachers of Mathematics, 1967, Arnold B. Chace, ed.) 1.1 Egypt 7 basic tables for addition, subtraction, and doubling and then a definite procedure for reducing multiplication and division problems into steps, each of which could be done using the tables. Besides the basic procedures of doubling, the Egyptian scribes used other techniques in performing arithmetic calculations. For example, they could find halves of numbers as well as multiply by 10; they could figure out what fractions had to be added to a given mixed number to get the next whole number; and they could determine by what fraction a given whole number needs to be multiplied to give a given fraction. These procedures are illustrated in problem 69 of the Rhind Papyrus, which includes the division of 80 by 3 2 and its subsequent check: 1 32 1 22 3 7 21 10 35 2 45 3 4 14 28 42 20 70 2 11 3 14 42 2 7 32 80 3 23 21 6 7 2 22 3 7 21 80 In the second line, the scribe took advantage of the decimal nature of his notation to give immediately the product of 3 2 by 10. In the fifth line, he used the 2/3 multiplication table mentioned earlier. The scribe then realized that since the numbers in the second column of the third through the fifth lines added to 79 3, he needed to add 2 and 6 in that column to get 80. Thus, because 6 × 3 2 = 21 and 2 × 3 2 = 7, it follows that 21 × 3 2 = 6 and 7 × 3 2 = 2, as indicated in the sixth and seventh lines. The check shows several uses of the table of division by 2 as well as great facility in addition. 1.1.2 Linear Equations and Proportional Reasoning The mathematical problems the scribes could solve, as illustrated in the Rhind and Moscow Papyri, deal with what we today call linear equations, proportions, and geometry. For example, the Egyptian papyri present two different procedures for dealing with linear equations. First, problem 19 of the Moscow Papyrus used our normal technique to find the number such that if it is taken 1 1/2 times and then 4 is added, the sum is 10. In modern notation, the equation is simply (1 1/2)x + 4 = 10. The scribe proceeded as follows: “Calculate the excess of this 10 over 4. The result is 6. You operate on 1 1/2 to find 1. The result is 2/3. You take 2/3 of this 6. The result is 4. Behold, 4 says it. You will find that this is correct.”4 Namely, after subtracting 4, the scribe noted that the reciprocal of 1 1/2 is 2/3 and then multiplies 6 by this quantity. Similarly, problem 35 of the Rhind Papyrus asked to find the size of a scoop that requires 3 1/3 trips to fill a 1 hekat measure. The scribe solved the equation, which would today be written as (3 1/3)x = 1 by dividing 1 by 3 1/3. He wrote the answer as 5 10 and proceeded to prove that the result is correct. 8 Chapter 1 Egypt and Mesopotamia The Egyptians’ more common technique of solving a linear equation, however, was what is usually called the method of false position, the method of assuming a convenient but probably incorrect answer and then adjusting it by using proportionality. For example, problem 26 of the Rhind Papyrus asked to find a quantity such that when it is added to 1/4 of itself the result is 15. The scribe’s solution was as follows: “Assume [the answer is] 4. Then 1 4 of 4 is 5. . . . Multiply 5 so as to get 15. The answer is 3. Multiply 3 by 4. The answer is 12.”5 In modern notation, the problem is to solve x + (1/4)x = 15. The first guess is 4, because 1/4 of 4 is an integer. But then the scribe noted that 4 + 1/4 . 4 = 5. To find the correct answer, he therefore multiplied 4 by the ratio of 15 to 5, namely, 3. The Rhind Papyrus has several similar problems, all solved using false position. The step-by-step procedure of the scribe can therefore be considered as an algorithm for the solution of a linear equation of this type. There is, however, no discussion of how the algorithm was discovered or why it works. But it is evident that the Egyptian scribes understood the basic idea of proportionality of two quantities. This understanding is further exemplified in the solution of more explicit proportion problems. For example, problem 75 asked for the number of loaves of pesu 30 that can be made from the same amount of flour as 155 loaves of pesu 20. (Pesu is the Egyptian measure for the inverse “strength” of bread and can be expressed as pesu = [number of loaves]/[number of hekats of grain], where a hekat is a dry measure approximately equal to 1/8 bushel.) The problem was thus to solve the proportion x/30 = 155/20. The scribe accomplished this by dividing 155 by 20 and multiplying the result by 30 to get 232 1/2. Similar problems occur elsewhere in the Rhind Papyrus and in the Moscow Papyrus. On the other hand, the method of false position is also used in the only quadratic equation extant in the Egyptian papyri. On the Berlin Papyrus, a small fragment dating from approximately the same time as the other papyri, is a problem asking to divide a square area of 100 square cubits into two other squares, where the ratio of the sides of the two squares is 1 to 3/4. The scribe began by assuming that in fact the sides of the two needed squares are 1 and 3/4, then calculated the sum of the areas of these two squares to be 12 + (3/4)2 = 1 9/16. But the desired sum of the areas is 100. The scribe realized that he could not compare areas directly but must compare their sides. So he took the square root of 1 9/16, namely, 1 41 , and compared this to the square root of 100, namely, 10. Since 10 is 8 times as large as 1 41 , the scribe concluded that the sides of the two other squares must be 8 times the original guesses, namely, 8 and 6 cubits, respectively. There are numerous more complicated problems in the extant papyri. For example, problem 64 of the Rhind Papyrus reads as follows: “If it is said to thee, divide 10 hekats of barley among 10 men so that the difference of each man and his neighbor in hekats of barley is 1/8, what is each man’s share?”6 It is understood in this problem, as in similar problems elsewhere in the papyrus, that the shares are to be in arithmetic progression. The average share is 1 hekat. The largest share could be found by adding 1/8 to this average share half the number of times as there are differences. However, since there is an odd number (9) of differences, 9 the scribe instead added half of the common difference (1/16) a total of 9 times to get 1 16 (1 2 16) as the largest share. He finished the problem by subtracting 1/8 from this value 9 times to get each share. A final problem, problem 23 of the Moscow Papyrus, is what we often think of today as a “work” problem: “Regarding the work of a shoemaker, if he is cutting out only, he can do 10 1.1 Egypt 9 pairs of sandals per day; but if he is decorating, he can do 5 per day. As for the number he can both cut and decorate in a day, what will that be?”7 Here the scribe noted that the shoemaker cuts 10 pairs of sandals in one day and decorates 10 pairs of sandals in two days, so that it takes three days for him to both cut and decorate 10 pairs. The scribe then divided 10 by 3 to find that the shoemaker can cut and decorate 3 1/3 pairs in one day. 1.1.3 Geometry As to geometry, the Egyptian scribes certainly knew how to calculate the areas of rectangles, triangles, and trapezoids by our normal methods. It is their calculation of the area of a circle, however, that is particularly interesting. Problem 50 of the Rhind Papyrus reads, “Example of a round field of diameter 9. What is the area? Take away 1/9 of the diameter; the remainder is 8. Multiply 8 times 8; it makes 64. Therefore, the area is 64.”8 In other words, the Egyptian scribe was using a procedure described by the formula A = (d − d/9)2 = [(8/9)d]2. A comparison with the formula A = (π/4)d 2 shows that the Egyptian value for the constant π in the case of area was 256/81 = 3.16049 . . . . Where did the Egyptians get this value, and why was the answer expressed as the square of (8/9)d rather than in modern terms as a multiple (here 64/81) of the square of the diameter? FIGURE 1.5 Octagon inscribed in a square of side 9, from problem 48 of the Rhind Mathematical Papyrus A hint is given by problem 48 of the same papyrus, in which is shown the figure of an octagon inscribed in a square of side 9 (Fig. 1.5). There is no statement of the problem, however, only a bare computation of 8 × 8 = 64 and 9 × 9 = 81. If the scribe had inscribed a circle in the same square, he would have seen that its area was approximately that of the octagon. What is the size of the octagon? It depends on how one interprets the diagram in the papyrus. If one believes the octagon to be formed by cutting off four corner triangles each having area 4 1/2, then the area of the octagon is 7/9 that of the square, namely, 63. The scribe therefore might have simply taken the area of the circle as A = (7/9)d 2 [= (63/81)d 2 ]. But since he wanted to find a square whose area was equal to the given circle, he may have approximated 63/81 by (8/9)2, thus giving the area of the circle in the form [(8/9)d]2 indicated in problem 50. On the other hand, in the diagram, the octagon does not look symmetric. So perhaps the octagon was formed by cutting off from the square of side 9 two diagonally opposite corner triangles each equal to 4 1/2 and two other corner triangles each equal to 4. This octagon then has area 64, as explicitly written on the papyrus, and thus this may be the square that the scribe wanted, which was equal in area to a circle. It should be noted that problem 50 is not an isolated problem of finding the area of a circle. In fact, there are several problems in the Rhind Papyrus where the scribe used the rule V = Bh to calculate the volume of a cylinder where B, the area of the base, is calculated by this circle rule. The scribes also knew how to calculate the volume of a rectangular box, given its length, width, and height. Because one of the prominent forms of building in Egypt was the pyramid, one might expect to find a formula for its volume. Unfortunately, such a formula does not appear in any extant document. The Rhind Papyrus does have several problems dealing with the seked (slope) of a pyramid; this is measured as so many horizontal units to one vertical unit rise. The workers building the pyramids, or at least their foremen, had to be aware of this value as they built. Since the seked is in effect the cotangent of the angle of slope of the pyramid’s faces, one can easily calculate the angles given the values appearing in the problems. It is 10 Chapter 1 Egypt and Mesopotamia not surprising that these calculated angles closely approximate the actual angles used in the construction of the three major pyramids at Giza. The Moscow Papyrus, however, does have a fascinating formula related to pyramids, namely, the formula for the volume of a truncated pyramid (problem 14): “If someone says to you: a truncated pyramid of 6 for the height by 4 on the base by 2 on the top, you are to square this 4; the result is 16. You are to double 4; the result is 8. You are to square this 2; the result is 4. You are to add the 16 and the 8 and the 4; the result is 28. You are to take 1/3 of 6; the result is 2. You are to take 28 two times; the result is 56. Behold, the volume is 56. You will find that this is correct.”9 If this algorithm is translated into a modern formula, with the length of the lower base denoted by a, that of the upper base by b, and the height by h, it gives the correct result V = h3 (a 2 + ab + b2). Although no papyrus gives the formula V = 31 a 2 h for a completed pyramid of square base a and height h, it is a simple matter to derive it from the given formula by simply putting b = 0. We therefore assume that the Egyptians were aware of this result. On the other hand, it takes a higher level of algebraic skill to derive the volume formula for the truncated pyramid from that for the complete pyramid. Still, although many ingenious suggestions involving dissection have been given, no one knows for sure how the Egyptians found their algorithm. No one knows either how the Egyptians found their procedure for determining the surface area of a hemisphere. But they succeeded in problem 10 of the Moscow Papyrus: “A basket with a mouth opening of 4 1/2 in good condition, oh let me know its surface area. First, calculate 1/9 of 9, since the basket is 1/2 of an egg-shell. The result is 1. Calculate the remainder as 8. Calculate 1/9 of 8. The result is 2/3 1/6 1/18 [that is, 8/9]. Calculate the remainder from these 8 after taking away those [8/9]. The result is 7 1/9. Reckon with 7 1/9 four and one-half times. The result is 32. Behold, this is its area. You will find that it is correct.”10 Evidently, the scribe calculated the surface area S of this basket of diameter d = 4 1/2 by first taking 8/9 of 2d, then taking 8/9 of the result, and finally multiplying by d. As a modern formula, this result would be S = 2( 89 d)2, or, since the area A of the circular opening of this hemispherical basket is given by A = ( 89 d)2 , we could rewrite this result as S = 2A, the correct answer. (It should be noted that there is not universal agreement that this calculation gives the area of a hemisphere. Some suggest that it gives the surface area of a half-cylinder.) 1.2 MESOPOTAMIA The Mesopotamian civilization is perhaps a bit older than the Egyptian, having developed in the Tigris and Euphrates River valley beginning sometime in the fifth millennium bce. Many different governments ruled this region over the centuries. Initially, there were many small city-states, but then the area was unified under a dynasty from Akkad, which lasted from approximately 2350 to 2150 bce. Shortly thereafter, the Third Dynasty of Ur rapidly expanded until it controlled most of southern Mesopotamia. This dynasty produced a very centralized bureaucratic state. In particular, it created a large system of scribal schools to train members of the bureaucracy. Although the Ur Dynasty collapsed around 2000 bce, the small city-states that succeeded it still demanded numerate scribes. By 1700 bce, Hammurapi, the 1.2 Mesopotamia 11 ruler of Babylon, one of these city-states, had expanded his rule to much of Mesopotamia and instituted a legal system to help regulate his empire (Fig. 1.6). FIGURE 1.6 Hammurapi on a stamp of Iraq FIGURE 1.7 Tablet from Uruk, c. 3200 bce, with number signs FIGURE 1.8 Babylonian clay tablet on a stamp of Austria Writing began in Mesopotamia, quite possibly in the southern city of Uruk, at about the same time as in Egypt, namely, at the end of the fourth millennium bce. In fact, writing began there also with the needs of accountancy, of the necessity of recording and managing labor and the flow of goods. The temple, the home of the city’s patron god or goddess, came to own large tracts of farming land and vast herds of sheep and goats. The scribes of the temple managed these assets to provide for the well-being of the god(dess) and his or her followers. Thus, in the temple of goddess Inana in Uruk, the scribes represented numbers on small clay slabs, using various pictograms to represent the objects that were being counted or measured. For example, five ovoids might represent five jars of oil. Or, as in the earliest known piece of school mathematics yet discovered, the scribe who wrote tablet W 19408,7611 used three different number signs to represent lengths as he calculated the area of a field (Fig. 1.7). Small circles represented 10 rods; a large D-shaped impression represented a unit of 60 rods, whereas a small circle within a large D represented 60 × 10 = 600 rods. On this tablet, there are two other signs, a horizontal line representing width and a vertical line representing length. The two widths of the quadrilateral field were each 2 × 600 = 1200, while the two lengths were 600 + 5 × 60 + 3 × 10 = 930 and 600 + 4 × 60 + 3 × 10 = 870. The approximate area could then be found by a standard ancient method of multiplying the average width by the average length; that is, A = ((w1 + w2)/2)((l1 + l2)/2). In this case, the answer was 1200 × 900 = 1,080,000. But since in the then current measurement system 1 square rod was equal to 1 sar, while 1800 sar were equal to 1 bur, the result here was 600 bur, a conspicuously “round” number, typical of answers in school tablets. On this particular tablet, as in other situations where quantities were measured, there were several different units of measure and different symbols for each type of unit. Here, the largest unit was equal to 60 of the smallest unit. This was typical in the units for many different types of objects, and at some time, the system of recording numbers developed to the point where the digit for 1 represented 60 as well. We do not know why the Mesopotamians decided to have one large unit represent 60 small units and then adapt this method for their numeration system. One plausible conjecture is that 60 is evenly divisible by many small integers. Therefore, fractional values of the “large” unit could easily be expressed as integral values of the “small.” But eventually, they did develop a sexagesimal (base-60) place value system, which in the third millennium bce became the standard system used throughout Mesopotamia. By that time, too, writing began to be used in a wide variety of contexts, all achieved by using a stylus on a moist clay tablet (Fig. 1.8). Thousands of these tablets have been excavated during the past 150 years. It was Henry Rawlinson (1810–1895) who, by the mid-1850s, was first able to translate this cuneiform writing by comparing the Persian and Mesopotamian cuneiform inscriptions of King Darius I of Persia (sixth century bce) on a rock face at Behistun (in modern Iran) describing a military victory. A large number of these tablets are mathematical in nature, containing mathematical problems and solutions or mathematical tables. Several hundreds of these have been copied, translated, and explained. These tablets, generally rectangular but occasionally round, usually fit comfortably into one’s hand and are an inch or so in thickness. Some, however, are as small as a postage stamp while others are as large as an encyclopedia volume. We are fortunate that these tablets are virtually indestructible, because they are our only source for Mesopotamian 12 Chapter 1 Egypt and Mesopotamia mathematics. The written tradition that they represent died out under Greek domination in the last centuries bce and was totally lost until the nineteenth century. The great majority of the excavated tablets date from the time of Hammurapi, while small collections date from the earliest beginnings of Mesopotamian civilization, from the centuries surrounding 1000 bce, and from the Seleucid period around 300 bce. Our discussion in this section, however, will generally deal with the mathematics of the “Old Babylonian” period (the time of Hammurapi), but, as is standard in the history of mathematics, we shall use the adjective “Babylonian” to refer to the civilization and culture of Mesopotamia, even though Babylon itself was the major city of the area for only a limited time. 1.2.1 Methods of Computation The Babylonians at various times used different systems of numbers, but the standardized system that the scribes generally used for calculations in the “Old Babylonian” period was a base-60 place value system together with a grouping system based on 10 to represent numbers up to 59. Thus, a vertical stylus stroke on a clay tablet represented 1 and a tilted stroke represented 10. By grouping they would, for example, represent 37 by . For numbers greater than 59, the Babylonians used a place value system; that is, the powers of 60, the base of this system, are represented by “places” rather than symbols, while the digit in each place represents the number of each power to be counted. Hence, 3 × 602 + 42 × 60 + 9 (or 13,329) was represented by the Babylonians as . (This will be written from now on as 3,42,09 rather than with the Babylonian strokes.) The Old Babylonians did not use a symbol for 0, but often left an internal space if a given number was missing a particular power. There would not be a space at the end of a number, making it difficult to distinguish 3 × 60 + 42 (3,42) from 3 × 602 + 42 × 60 (3,42,00). Sometimes, however, they would give an indication of the absolute size of a number by writing an appropriate word, typically a metrological one, after the numeral. Thus, “3 42 sixty” would represent 3,42, while “3 42 thirty-six hundred” would mean 3,42,00. On the other hand, the Babylonians never used a symbol to represent zero in the context of “nothingness,” as in our 42 − 42 = 0. That the Babylonians used tables in the process of performing arithmetic computations is proved by extensive direct evidence. Many of the preserved tablets are in fact multiplication tables. No addition tables have turned up, however. Because over 200 Babylonian table texts have been analyzed, it may be assumed that these did not exist and that the scribes knew their addition procedures well enough so they could write down the answers when needed. On the other hand, there are many examples of “scratch tablets,” on which a scribe has performed various calculations in the process of solving a problem. In any case, since the Babylonian 1.2 Mesopotamia 13 number system was a place value system, the actual algorithms for addition and subtraction, including carrying and borrowing, may well have been similar to modern ones. For example, to add 23,37 (= 1417) to 41,32 (= 2492), one first adds 37 and 32 to get 1,09 (= 69). One writes down 09 and carries 1 to the next column. Then 23 + 41 + 1 = 1, 05 (= 65), and the final result is 1,05,09 (= 3909). Because the place value system was based on 60, the multiplication tables were extensive. Any given one listed the multiples of a particular number, say, 9, from 1 × 9 to 20 × 9 and then gave 30 × 9, 40 × 9, and 50 × 9 (Fig. 1.9). If one needed the product 34 × 9, one simply added the two results 30 × 9 = 4, 30 (= 270) and 4 × 9 = 36 to get 5,06 (= 306). For multiplication of two- or three-digit sexagesimal numbers, one needed to use several such tables. The exact algorithm the Babylonians used for such multiplications—where the partial products are written and how the final result is obtained—is not known, but it may well have been similar to our own. One might think that for a complete system of tables, the Babylonians would have one for each integer from 2 to 59. Such was not the case, however. In fact, although there are no tables FIGURE 1.9 A Babylonian multiplication table for 9 (Department of Archaeology, University of Pennsylvania) 14 Chapter 1 Egypt and Mesopotamia for 11, 13, 17, for example, there are tables for 1,15, 3,45, and 44,26,40. We do not know precisely why the Babylonians made these choices; we do know, however, that, with the single exception of 7, all multiplication tables so far found are for regular sexagesimal numbers, that is, numbers whose reciprocal is a terminating sexagesimal fraction. The Babylonians treated all fractions as sexagesimal fractions, analogous to our use of decimal fractions. Namely, the first place after the “sexagesimal point” (which we denote by “;”) represents 60ths, the next place 3600ths, and so on. Thus, the reciprocal of 48 is the sexagesimal fraction 0;1,15, which represents 1/60 + 15/602, while the reciprocal of 1,21 (= 81) is 0;0,44,26,40, or 44/602 + 26/603 + 40/604. Because the Babylonians did not indicate an initial 0 or the sexagesimal point, this last number would just be written as 44,26,40. As noted, there exist multiplication tables for this regular number. In such a table there is no indication of the absolute size of the number, nor is one necessary. When the Babylonians used the table, of course, they realized that, as in today’s decimal calculations, the eventual placement of the sexagesimal point depended on the absolute size of the numbers involved, and this placement was then done by context. Besides multiplication tables, there are also extensive tables of reciprocals, one of which is in part reproduced here. A table of reciprocals is a list of pairs of numbers whose product is 1 (where the 1 can represent any power of 60). Like the multiplication tables, these tables only contained regular sexagesimal numbers. 2 3 10 30 20 6 16 3, 45 25 2, 24 40 1, 30 48 1, 15 1, 04 56, 15 1, 21 44, 26, 40 The reciprocal tables were used in conjunction with the multiplication tables to do division. Thus, the multiplication table for 1,30 (= 90) served not only to give multiples of that number but also, since 40 is the reciprocal of 1,30, to do divisions by 40. In other words, the Babylonians considered the problem 50 ÷ 40 to be equivalent to 50 × 1/40, or in sexagesimal notation, to 50 × 0;1,30. The multiplication table for 1,30, part of which appears here, then gives 1,15 (or 1,15,00) as the product. The appropriate placement of the sexagesimal point gives 1;15(= 1 1/4) as the correct answer to the division problem. 1 1,30 2 3 3 4,30 10 15 11 16,30 12 18 30 40 50 45 1 1,15 1.2.2 Geometry The Babylonians had a wide range of problems to which they applied their sexagesimal place value system. For example, they developed procedures for determining areas and volumes of various kinds of figures. They worked out algorithms to determine square roots. They solved problems that we would interpret in terms of linear and quadratic equations, problems often related to agriculture or building. In fact, the mathematical tablets themselves are generally concerned with the solution of problems, to which various mathematical techniques are applied. So we will look at some of the problems the Babylonians solved and try to figure out what lies behind their methods. In particular, we will see that the reasons behind many of the Babylonian procedures come from a tradition different from the accountancy traditions 1.2 Mesopotamia 15 with which Babylonian mathematics began. This second tradition was the “cut-and-paste” geometry of the surveyors, who had to measure fields and lay out public works projects. As we will see, these manipulations of squares and rectangles not only developed into procedures for determining square roots and finding Pythagorean triples, but they also developed into what we can think of as “algebra.” As we work through the Babylonian problems, we must keep in mind that, like the Egyptians, the scribes did not have any symbolism for operations or unknowns. Thus, solutions are presented with purely verbal techniques. We must also remember that the Babylonians often thought about problems in ways different from the ways we do. Thus, even though their methods are usually correct, they may seem strange to us. As one example of the scribes’ different methods, we consider their procedures for determining lengths and areas. In general, in place of our formulas for calculating such quantities, they presented coefficient lists, lists of constants that embody mathematical relationships between certain aspects of various geometrical figures. Thus, the number 0;52,30 (= 7/8) as the coefficient for the height of a triangle means that the altitude of an equilateral triangle is 7/8 of the base, while the number 0;26,15 (= 7/16) as the coefficient for area means that the area of an equilateral triangle is 7/16 times the square of a side. (Note, √ of course, that these results are only approximately correct, in that they both approximate 3 by 7/4.) In each case, the idea is that the “defining component” for the triangle is the side. We too would use the length of a side as the defining component for an equilateral triangle. But for a circle, we generally use the radius r as that component and therefore give formulas for the circumference and area in terms of r. The Babylonians, on the other hand, took the circumference as the defining component of a circle. Thus, they gave two coefficients for the circle: 0;20 (= 1/3) for the diameter and 0;05 (= 1/12) for the area. The first coefficient means that the diameter is one-third of the circumference, while the second means that the area is one-twelfth of the square of the circumference. For example, on the tablet YBC 7302, there is a circle with the numbers 3 and 9 written on the outside and the number 45 written on the inside (Fig. 1.10). The interpretation of this is that the circle has circumference 3 and that the area is found by dividing 9 = 32 by 12 to get 0;45 (= 3/4). Another tablet, Haddad 104, illustrates that circle calculations virtually always use the circumference. On this tablet, there is a problem asking to find the area of the cross section of a log of diameter 1;40 (= 1 23 ). Rather than determine the radius, the scribe first multiplies by 3 to find that the circumference FIGURE 1.10 Tablet YBC 7302 illustrating measurements on a circle 3 45 9 16 Chapter 1 Egypt and Mesopotamia FIGURE 1.11 Babylonian barge and bull’s-eye FIGURE 1.12 Babylonian concave square 1 is equal to 5, then squares 5 and multiplies by 1/12 to get the area 2;05 (= 2 12 ). Note further, of course, that the Babylonian value for what we denote as π , the ratio of circumference to diameter, is 3; this value produces the value 4π = 12 as the constant by which to divide the square of the circumference to give the area. There are also Babylonian coefficients for other figures bounded by circular arcs. For example, the Babylonians calculated areas of two different double bows: the “barge,” made up of two quarter-circle arcs, and the “bull’s-eye,” composed of two third-circle arcs (Fig. 1.11). In analogy with the circle, the defining component of these figures was the arc making up one side. The coefficient of the area of the barge is 0;13,20 (= 2/9), while that of the bull’seye is 0;16,52,30 (= 9/32). Thus, the areas of these two figures are calculated as (2/9)a 2 and (9/32)a 2, respectively, where in each case a is the length of that arc. These results are accurate √ under the assumptions that the area of the circle is C 2/12 and that 3 = 7/4. Similarly, the coefficient of the area of the concave square (Fig. 1.12) is 0;26,40 (= 4/9), where the defining component is one of the four quarter-circle arcs forming the boundary of the region.12 Clearly, the use of these coefficients shows that the scribes recognized that lengths of particular lines in given figures were proportional to the length of the defining component, while the area was proportional to the square of that component. The Babylonians also dealt with volumes of solids. They realized that the volume V of a rectangular block is V = wh, and they also knew how to calculate the volume of prisms given the area of the base. But just like in Egypt, there is no document that explicitly gives the volume of a pyramid, even though the Babylonians certainly built pyramidal structures. Nevertheless, on tablet BM 96954, there are several problems involving a grain pile in the shape of a rectangular pyramid with an elongated apex, like a pitched roof (Fig. 1.13). The method of solution corresponds to the modern formula hw t V = + , 3 2 where is the length of the solid, w the width, h the height, and t the length of the apex. Although no derivation of this correct formula is given on the tablet, we can derive it by breaking up the solid into a triangular prism with half a rectangular pyramid on each side. Then the volume would be the sum of the volumes of these solids (Fig. 1.14). Thus, V = volume of triangular prism + volume of rectangular pyramid, or hw( − t) hw hwt hw t hwt + = + = + , V = 2 3 3 6 3 2 as desired.13 It therefore seems reasonable to assume from the result discussed here that the Babylonians were aware of the correct formula for the volume of a pyramid. 1.2 Mesopotamia 17 t FIGURE 1.13 Babylonian grain pile h w FIGURE 1.14 Dissection of grain pile h w h t −t w That assumption is even more convincing because there is a tablet giving a correct formula for the volume of a truncated pyramid with square base a 2 , square top b2, and height h in 1 a−b 2 2 the form V = [( a+b 2 ) + 3 ( 2 ) ]h. The complete pyramid formula, of course, follows from this by putting b = 0. On the other hand, there are tablets where this volume is calculated by the rule V = 21 (a 2 + b2)h, a simple but incorrect generalization of the rule for the area of the trapezoid. It is well to remember, however, that although this formula is incorrect, the calculated answers would not be very different from the correct ones. It is difficult to see how anyone would realize that the answers were wrong in any case, because there was no accurate method for measuring the volume empirically. However, because the problems in which these formulas occurred were practical ones, often related to the number of workmen needed to build a particular structure, the slight inaccuracy produced by using this rule would have little effect on the final answer. 1.2.3 Square Roots and the Pythagorean Theorem We next consider another type of Babylonian algorithm, the square root algorithm. Usually, when square roots are needed in solving problems, the problems are arranged so that the square root is one that is listed in a table of square roots, of which many exist, and is a rational number. But there are cases where an irrational square root is needed, in particular, 18 Chapter 1 Egypt and Mesopotamia √ 5 2. When this particular value occurs, the result is generally written as 1;25 (= 1 12 ). There is, however, an interesting tablet, YBC 7289, on which is drawn a square with side indicated as 30 and two numbers, 1:24,51,10 and 42;25,35, written on the diagonal (Fig. 1.15). The product of 30 by 1;24,51,10 is precisely 42;25,35. It is then a reasonable assumption that √the last number represents the length of the diagonal and that the other number represents 2. FIGURE 1.15 Tablet YBC 7289 with the square root of 2 √ Whether 2 is given as 1;25 or as 1;24,51,10, there is no record as to how the value was calculated. But because the scribes were surely aware that the square of neither of these was exactly 2, or that these values were not exactly the length of the side of a square of area 2, they must have known that these values were approximations. How were they determined? One possible method, a method for which there is some textual evidence, begins with the algebraic identity (x + y)2 = x 2 + 2xy + y 2, whose validity was probably discovered by the Babylonians from √ its geometric equivalent. Now given a square of area N for which one wants the side N, the first step would be to choose a regular value a close to, but less than, the desired result. Setting b = N − a 2 , the next step is to find c so that 2ac + c2 is as close as possible to b (Fig. 1.16). If a 2 is “close enough” to N√, then √ c2 will be small in relation to 2ac, so c can be chosen to equal (1/2)b(1/a), that is, N = a 2 + b ≈ a + (1/2)b(1/a). (In keeping with Babylonian methods, the value for c has been written as a product rather than a quotient, and, since one of the factors √ is the reciprocal of a, we see why a must a 2 − b ≈ a − (1/2)b(1/a). In the particular be regular.) A similar argument shows that √ 2 case of √2, one √ begins with a = 1;20 (= 4/3). Then a = 1;46,40, b = 0;13,20, and 1/a = 0;45, so 2 = 1;46,40 + 0;13,20 ≈ 1;20 + (0;30)(0;13,20)(0;45) = 1;20 + 0;05 = 1;25 (or 17/12). To calculate the better approximation 1;24,51,10, one would have to repeat this procedure, with a = 1;25. Unfortunately, 1;25 is not a regular sexagesimal number. The scribes could, however, have found an approximation to the reciprocal, say, 0;42,21,10, and then calculated √ 2 = 1;252 − 0;00,25 ≈ 1;25 − 0;30 × 0;00,25 × 0;42,21,10 = 1;24,51,10,35,25. Because the approximation formula leads to a slight overestimate of the true value, the scribes would have truncated this answer to the desired 1;24,51,10. There is, however, no direct 1.2 Mesopotamia 19 FIGURE 1.16 √ Geometric version of N = √ 1 . . 1 2 a +b≈a+ 2 b a ac ac c2 ac ⎧ ⎪ ⎪ ⎪ ⎪ ⎪ ⎪ ⎪ ⎪ ⎪ ⎪ ⎪ ⎨ ⎪ ⎪ ⎪ ⎪ ⎪ ⎪ ⎪ ⎪ ⎪ ⎪ ⎪ ⎩ a2 b evidence of this calculation nor even any evidence for the use of more than one step of this approximation procedure. One of the Babylonian square root problems was connected to the relation between the side of a square and its diagonal. That relation is a special case of the result known as the Pythagorean Theorem: In any right triangle, the sum of the areas of the squares on the legs equals the area of the square on the hypotenuse. This theorem, named after the sixth-century bce Greek philosopher-mathematician, is arguably the most important elementary theorem in mathematics, since its consequences and generalizations have wide-ranging application. Nevertheless, it is one of the earliest theorems known to ancient civilizations. In fact, there is evidence that it was known at least 1000 years before Pythagoras. In particular, there is substantial evidence of interest in Pythagorean triples, triples of integers (a, b, c) such that a 2 + b2 = c2, in the Babylonian tablet Plimpton 322 (Fig. 1.17).14 The extant piece of the tablet consists of four columns of numbers. Other columns were probably broken off on the left. The numbers on the tablet are shown in Table 1.1, reproduced in modern decimal notation with the few corrections that recent editors have made and with one extra column, y (not on the tablet), added on the right. It was a major piece of mathematical detective work for modern scholars, first, to decide that this was a mathematical work rather than a list of orders from a pottery business and, second, to find a reasonable mathematical explanation. But find one they did. The columns headed x and d (whose headings in the original can be translated as “square-side of the short side” and “squareside of the diagonal”) contain in each row two of the three numbers of a Pythagorean triple. It is easy enough to subtract the square of column x from the square of column d. In each case a perfect square results, whose square root is indicated in the added column, y. Finally, the first column on the left represents the quotient ( dy )2. How and why were these triples derived? One cannot find Pythagorean triples of this size by trial and error. There have been many suggestions over the years as to how the scribe found these as well as to the purpose of the tablet. If one considers this question as purely a mathematical one, there are many methods that would work to generate the table. But since this tablet was written at a particular time and place, probably in Larsa around 1800 bce, an understanding of its construction and meaning must come from an understanding of the context of the time and how mathematical tablets were generally written. In particular, it is important to note that the first column in a Babylonian table is virtually always written in numerical order (either ascending or descending), while subsequent columns depend on those to their left. Unfortunately, in this instance it is believed that the initial columns 20 Chapter 1 Egypt and Mesopotamia FIGURE 1.17 Plimpton 322 (Source: George Arthur Plimpton Collection, Rare Book and Manuscript Library, Columbia University) TABLE 1.1 Numbers on the Babylonian tablet Plimpton 322, reproduced in modern decimal notation. (The column to the right, labeled y, does not appear on the tablet.) 2 d x d # y y 1.9834028 1.9491586 1.9188021 1.8862479 1.8150077 1.7851929 1.7199837 1.6845877 1.6426694 1.5861226 1.5625 1.4894168 1.4500174 1.4302388 1.3871605 119 3367 4601 12,709 65 319 2291 799 481 4961 45 1679 161 1771 28 169 4825 6649 18,541 97 481 3541 1249 769 8161 75 2929 289 3229 53 1 2 3 4 5 6 7 8 9 10 11 12 13 14 15 120 3456 4800 13,500 72 360 2700 960 600 6480 60 2400 240 2700 45 1.2 Mesopotamia 21 on the left are missing. However, some clues as to the meaning of the table reside in the words at the top of the column we have labeled ( dy )2 . Deciphering the words was difficult because some of the cuneiform wedges were damaged, but it appears that the heading means “the holding-square of the diagonal from which 1 is torn out so that the short side comes up.” The “1” in that heading indicates that the author is dealing with reciprocal pairs, very common in Babylonian tables. To relate reciprocals to Pythagorean triples, we note that to find integer solutions to the equation x 2 + y 2 = d 2, one can divide by y and first find solutions to ( xy )2 + 1 = ( dy )2 or, setting u = xy and v = dy , to u2 + 1 = v 2. This latter equation is equivalent to (v + u)(v − u) = 1. That is, we can think of v + u and v − u as the sides of a rectangle whose area is 1 (Fig. 1.18). Now split off from this rectangle one with sides u and v − u and move it to the bottom left after a rotation of 90◦. The resulting figure is an L-shaped figure, usually called a gnomon, with long sides both equal to v, a figure that is the difference v 2 − u2 = 1 of two squares. Note that the larger square is the square on the diagonal of the right triangle with sides (u, 1, v). The area of that square, v 2 = (d/y)2, is the entry in the leftmost column on the extant tablet, and furthermore, that square has a gnomon of area 1 torn out so that the remaining square is the square on the short side of the right triangle, as the column heading actually says. vu FIGURE 1.18 A rectangle of area 1 turned into the difference of two squares vu vu u v u u u To calculate the entries on the tablet, it is possible that the author began with a value for what we have called v + u. Next, he found its reciprocal v − u in a table and solved for u = 21 [(v + u) − (v − u)]. The first column in the table is then the value 1 + u2. He could then find v by taking the square root of 1 + u2. Since (u, 1, v) satisfies the Pythagorean identity, the author could find a corresponding integral Pythagorean triple by multiplying each of these values by a suitable number y, one chosen to eliminate “fractional” values. For example, if v + u = 2;15 (= 2 41 ), the reciprocal v − u is 0;26,40 (= 4/9). We then find u = 0;54,10 = 65/72. find v by taking half the sum √ We would √ of v + u√and v − u, but our √ 25 scribe found v as 1 + u2 = 1;48,54,01,40 = 1;20,50, or 1 + u2 = 1.8150077 = 1 72 . Multiplying the values for u, v, and 1 by 1,12 = 72 gives the values 65 and 97 for x and d, respectively, shown in line 5 of the table, as well as the value 72 for y. Conversely, the value of v + u for line 1 of the table can be found by adding 169/120 (= 1;24,30) and 119/120 (= 0;59,30) to get 288/120 (= 2;24). Why were the particular Pythagorean triples on this tablet chosen? Again, we cannot know the answer definitively. But if we calculate the values of v + u for every line of the tablet, we notice that they form a decreasing sequence of regular sexagesimal numbers of no more than 22 Chapter 1 ⎧ ⎪ ⎪ ⎪ ⎪ ⎪ 40 ⎨ ⎪ A ⎪ ⎪ ⎪ ⎪ ⎩ B Egypt and Mesopotamia r 30 C FIGURE 1.19 Circumscribing a circle about an isosceles triangle four places from 2;24 to 1;48. Not all such numbers are included—there are five missing— but it is possible that the scribe may have decided that the table was long enough without them. He may also have begun with numbers larger than 2;24 or continued with numbers smaller than 1;48 on tablets that have not yet been unearthed. In any case, it is likely that this column of values for v + u, in descending numerical order, was one of the missing columns on the original tablet. And our author, quite probably a teacher, had thus worked out a list of integral Pythagorean triples, triples that could be used in constructing problems for students for which he would know that the solution would be possible in integers or finite sexagesimal fractions. Whether or not the method presented above was the one the Babylonian scribe used to write Plimpton 322, the fact remains that the scribes were well aware of the Pythagorean relationship. And although this particular table offers no indication of a geometrical relationship except for the headings of the columns, there are problems in Old Babylonian tablets making explicit geometrical use of the Pythagorean Theorem. For example, in a problem from tablet BM 85196, a beam of length 30 stands against a wall. The upper end has slipped down a distance 6. How far did the lower end move? Namely, d = 30√and y = 24 are√given, and x is to be found. The scribe calculated x using the theorem: x = 302 − 242 = 324 = 18. Another slightly more complicated example comes from tablet TMS 1 found at Susa in modern Iran. The problem is to calculate the radius of a circle circumscribed about an isosceles triangle with altitude 40 and base 60. Applying the Pythagorean theorem to the right triangle ABC (Fig. 1.19), whose hypotenuse is the desired radius, gives the relationship r 2 = 302 + (40 − r)2 . This could be easily transformed into (1, 20)(r − 20) = 15,00 and then, by multiplying by the reciprocal 0;0,45 of 1,20, into r − 20 = (0;0,45)(15,00) = 11;15, from which the scribe found that r = 31;15. 1.2.4 Solving Equations The previous problem involved what we would call the solution of an equation. Such problems were very frequent on the Babylonian tablets. Linear equations of the form ax = b are generally solved by multiplying each side by the reciprocal of a. (Such equations often occur, as in the previous example, in the process of solving a complex problem.) In more complicated situations, such as systems of two linear equations, the Babylonians, like the Egyptians, used the method of false position. Here is an example from the Old Babylonian text VAT 8389: One of two fields yields 2/3 sila per sar, the second yields 1/2 sila per sar, where sila and sar are measures for capacity and area, respectively. The yield of the first field was 500 sila more than that of the second; the areas of the two fields were together 1800 sar. How large is each field? It is easy enough to translate the problem into a system of two equations with x and y representing the unknown areas: 1 2 x − y = 500 3 2 x + y = 1800 A modern solution might be to solve the second equation for x and substitute the result in the first. But the Babylonian scribe here made the initial assumption that x and y were both 1.2 Mesopotamia 23 equal to 900. He then calculated that (2/3) . 900 − (1/2) . 900 = 150. The difference between the desired 500 and the calculated 150 is 350. To adjust the answers, the scribe presumably realized that every unit increase in the value of x and consequent unit decrease in the value of y gave an increase in the “function” (2/3)x − (1/2)y of 2/3 + 1/2 = 7/6. He therefore needed only to solve the equation (7/6)s = 350 to get the necessary increase s = 300. Adding 300 to 900 gave him 1200 for x while subtracting gave him 600 for y, the correct answers. Presumably, the Babylonians also solved complex single linear equations by false position, although the few such problems available do not reveal their method. For example, here is a problem from tablet YBC 4652: “I found a stone, but did not weigh it; after I added one-seventh and then one-eleventh [of the total], it weighed 1 mina [= 60 gin]. What was the original weight of the stone?”15 We can translate this into the modern equation (x + x/7) + 1/11(x + x/7) = 60. On the tablet, the scribe just presented the answer, here x = 48 81 . If he had solved the problem by false position, the scribe would first have guessed that y = x + x/7 = 11. Since then y + (1/11)y = 12 instead of 60, the guess must be increased by the factor 60/12 = 5 to the value 55. Then, to solve x + x/7 = 55, the scribe could have guessed x = 7. This value would produce 7 + 7/7 = 8 instead of 55. So the last step would be to multiply the guess of 7 by the factor 55/8 to get 385/8 = 48 81 , the correct answer. While tablets containing explicit linear problems are limited, there are very many Babylonian tablets whose problems can be translated into quadratic equations. In fact, many Old Babylonian tablets contain extensive lists of quadratic problems. And in solving these problems, the scribes made full use of the “cut-and-paste” geometry developed by the surveyors. In particular, they applied this to various standard problems such as finding the length and width of a rectangle, given the semiperimeter and the area. For example, consider the problem x + y = 6 21 , xy = 7 21 from tablet YBC 4663. The scribe first halved 6 21 to get 3 41 . 9 1 . From this is subtracted 7 21 , leaving 3 16 , and then the Next he squared 3 41 , getting 10 16 3 1 3 square root is extracted to get 1 4 . The length is thus 3 4 + 1 4 = 5, while the width is given as 3 41 − 1 43 = 1 21 . A close reading of the wording of the tablets indicates that the scribe had in mind a geometric procedure (Fig. 1.20), where for the sake of generality the sides have been labeled in accordance with the generic system x + y = b, xy = c. The scribe began by x−y halving the sum b and then constructing the square on it. Since b/2 = x − x−y 2 =y+ 2 , x−y the square on b/2 exceeds the original rectangle of area c by the square on 2 ; that is, x+y 2 2 = xy + x−y 2 2 . The figure then shows that if one adds the side of this square, namely, (b/2)2 − c, to b/2, one finds the length x, while if one subtracts it from b/2, one gets the width y. The algorithm is therefore expressible in the form b b x = + (b/2)2 − c y = − (b/2)2 − c. 2 2 24 Chapter 1 Egypt and Mesopotamia x FIGURE 1.20 y ⎧ ⎪ ⎪ ⎪ ⎪ ⎪ ⎨ ⎪ ⎪ ⎪ ⎪ ⎪ ⎩ ⎧ ⎪ ⎪ ⎪ ⎪ ⎪ ⎪ ⎨ ⎪ ⎪ ⎪ ⎪ ⎪ ⎪ ⎩ ⎧ ⎪ ⎪ ⎪ ⎪ ⎪ ⎨ ⎪ ⎪ ⎪ ⎪ ⎪ ⎩ ⎧ ⎪ ⎪ ⎪ ⎪ ⎪ b/2 ⎨ ⎪ ⎪ ⎪ ⎪ ⎪ ⎩ ⎧ ⎪ ⎪ ⎪ ⎪ ⎪ ⎨ ⎪ ⎪ ⎪ ⎪ ⎪ ⎩ Geometric procedure for solving the system x + y = b, xy = c b/2 xy 2 y xy 2 xy 2 Geometry is also at the base of the Babylonian solution of what we would consider a single quadratic equation. Several such problems are given on tablet BM 13901, including the following, where the translation shows the geometric flavor of the problem: “I summed the area and two-thirds of my square-side and it was 0;35. You put down 1, the projection. Two-thirds of 1, the projection, is 0;40. You combined its half, 0;20 and 0;20. You add 0;06,40 to 0;35 and 0;41,40 squares 0;50. You take away 0;20 that you combined from the middle of 0;50 and the square-side is 0;30.”16 In modern terms, the equation to be solved is x 2 + (2/3)x = 7/12. At first glance, it would appear that the statement of the problem is not a geometric one, since we are asked to add a multiple of a side to an area. But the word “projection” indicates that this two-thirds multiple of a side is to be considered as two-thirds of the rectangle of length 1 and unknown side x. For the solution, the scribe took half of 2/3 and squared it (“combine its half, 0;20 and 0;20”), then took the result 1/9 (or 0;06,40) and added it to 7/12 (0;35) to get 25/36 (0;41,40). The scribe then noted that 5/6 (0;50) is the square root of 25/36 (“0;41,40 squares 0;50”). He then subtracted the 1/3 from 5/6 to get the result 1/2 (“the square-side is 0;30”). The Babylonian rule exemplified by this problem is easily transl…
Collepals.com Plagiarism Free Papers
Are you looking for custom essay writing service or even dissertation writing services? Just request for our write my paper service, and we'll match you with the best essay writer in your subject! With an exceptional team of professional academic experts in a wide range of subjects, we can guarantee you an unrivaled quality of custom-written papers.
Get ZERO PLAGIARISM, HUMAN WRITTEN ESSAYS
Why Hire Collepals.com writers to do your paper?
Quality- We are experienced and have access to ample research materials.
We write plagiarism Free Content
Confidential- We never share or sell your personal information to third parties.
Support-Chat with us today! We are always waiting to answer all your questions.
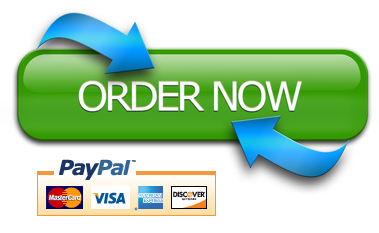