As you learned this week, milestones play an important role in the early years of a childs development. While we know that children grow and develop at their own pace, milestones show us that a child is developing as expected. This week, you will continue to support Paul and Amy and introduce them to an important app
As you learned this week, milestones play an important role in the early years of a child’s development. While we know that children grow and develop at their own pace, milestones show us that a child is developing as expected. This week, you will continue to support Paul and Amy and introduce them to an important app.
To prepare for this discussion,
· Read Chapter 5: Physical Development in Infancy and Toddlerhood
· Watch To Walk CDE 119 Full Links to an external site.
· Download Android’s CDC Milestone Tracker Links to an external site. or iPhone’s CDC’s Milestone Tracker Links to an external site. .
· Set up a profile in your CDC Milestone App by using Navigating the CDC Milestone Tracker App guide Links to an external site. .
For this discussion, you will utilize the case study below:
Case Study Paul and Amy have been loving the first two months of parenthood with their daughter, Charlie. At Charlie’s two-month well-visit, they asked many questions about Charlie’s physical development. Their pediatrician suggested that Paul and Amy download the CDC milestone tracker app so they could keep track of Charlie’s development and see how she is doing with mastering milestones. |
In your initial post, assume the role of Paul or Amy and provide a review of the CDC Milestone Tracker app that includes the following:
· Rate how you like using the app on a scale of 1 to 5 (one being you would not recommend and a five being a superior rating). Provide at least two reasons to support your rating.
· Explain at least two elements of the app that you think are helpful for tracking physical development from 2 months to 2 years.
· Discuss how you might use this app to ensure you are providing developmentally appropriate learning activities for children from 2 months to 2 years.
,
CHAPTER 5 PHYSICAL DEVELOPMENT IN INFANCY AND TODDLERHOOD
Mother
Shang Meng Lei, 9 years, China
This painting captures a mother and her infant affectionately imitating each other’s facial expressions and gestures. During the first year, infants grow rapidly, move on their own, increasingly investigate and make sense of their surroundings, and learn from others.
Reprinted with permission from The International Museum of Children’s Art, Oslo, Norway
WHAT’S AHEAD IN CHAPTER 5
5.1 Body Growth
Changes in Body Size and Muscle–Fat Makeup • Changes in Body Proportions • Individual and Group Differences
5.2 Brain Development
Development of Neurons • Measures of Brain Functioning • Development of the Cerebral Cortex • Sensitive Periods in Brain Development • Changing States of Arousal
■ Biology and Environment: Brain Plasticity: Insights from Research on Children with Brain Injury
■ Cultural Influences: Cultural Variation in Infant Sleeping Arrangements
5.3 Influences on Early Physical Growth
Heredity • Nutrition • Malnutrition • Emotional Well-Being
■ Social Issues: Health: Lead Exposure and Children’s Development
5.4 Learning Capacities
Classical Conditioning • Operant Conditioning • Habituation • Statistical Learning • Imitation
5.5 Motor Development
The Sequence of Motor Development • Motor Skills as Dynamic Systems • Fine-Motor Development: Reaching and Grasping
5.6 Perceptual Development
Hearing • Vision • Object Perception • Intermodal Perception • Understanding Perceptual Development
■ Biology and Environment: “Tuning in” to Familiar Speech, Faces, and Music: A Sensitive Period for Culture-Specific Learning
On a brilliant June morning, 16-month-old Caitlin emerged from her front door, ready for the short drive to the child-care home where she spent her weekdays while her mother, Carolyn, and her father, David, worked. Clutching a teddy bear in one hand and her mother’s arm with the other, Caitlin descended the steps. “One! Two! Threeeee!” Carolyn counted as she helped Caitlin down. “How much she’s changed!” Carolyn thought to herself, looking at the child who, not long ago, had been a newborn. With her first steps, Caitlin had passed from infancy to toddlerhood—a period spanning the second year of life. At first, Caitlin did, indeed, “toddle” with an awkward gait, tipping over frequently. But her face reflected the thrill of conquering a new skill.
As they walked toward the car, Carolyn and Caitlin spotted 3-year-old Eli and his father, Kevin, in the neighboring yard. Eli dashed toward them, waving a bright yellow envelope. Carolyn bent down to open the envelope and took out a card. It read, “Announcing the arrival of Grace Ann. Born: Cambodia. Age: 16 months.” Carolyn turned toward Kevin and Eli. “That’s wonderful news! When can we see her?”
“Let’s wait a few days,” Kevin suggested. “Monica’s taken Grace to the doctor this morning. She’s underweight and malnourished.” Kevin described Monica’s first night with Grace in a hotel room in Phnom Penh. Grace lay on the bed, withdrawn and fearful. Eventually she fell asleep, gripping crackers in both hands.
Carolyn felt Caitlin’s impatient tug at her sleeve. Off they drove to child care, where Vanessa had just dropped off her 18-month-old son, Timmy. Within moments, Caitlin and Timmy were in the sandbox, shoveling sand into plastic cups and buckets with the help of their caregiver, Ginette.
A few weeks later, Grace joined Caitlin and Timmy at Ginette’s child-care home. Although still unable to crawl or walk, she had grown taller and heavier, and her sad, vacant gaze had given way to an alert expression, a ready smile, and an enthusiastic desire to imitate and explore. When Caitlin headed for the sandbox, Grace stretched out her arms, asking Ginette to carry her there, too. Soon Grace was pulling herself up at every opportunity. Finally, at age 18 months, she walked!
This chapter traces physical growth during the first two years—one of the most remarkable and busiest times of development. We will see how rapid changes in the infant’s body and brain support learning, motor skills, and perceptual capacities. Caitlin, Grace, and Timmy will join us along the way to illustrate how individual differences and environmental influences affect physical development. ■
5.1 BODY GROWTH
5.1 Describe major changes in body growth over the first two years.
The next time you’re walking in your neighborhood park or at the mall, note the contrast between infants’ and toddlers’ physical capabilities. One reason for the vast changes in what children can do over the first two years is that their bodies change enormously—faster than at any other time after birth.
5.1.1 Changes in Body Size and Muscle–Fat Makeup
By the end of the first year, a typical infant’s height is about 32 inches—more than 50 percent greater than at birth. By 2 years, it is 75 percent greater, averaging 36 inches. Similarly, by age 5 months, birth weight has doubled to about 15 pounds. At 1 year it has tripled to 22 pounds, and at 2 years it has quadrupled to about 30 pounds.
Figure 5.1 illustrates this dramatic increase in body size. But rather than making steady gains, infants and toddlers grow in little spurts. In one study, children who were followed over the first 21 months of life went for periods of 7 to 63 days with no growth, then added as much as half an inch in a 24-hour period! Almost always, parents described their babies as irritable, very hungry, and sleeping more on the days before a spurt (Lampl, 1993; Lampl & Johnson, 2011).
One of the most obvious changes in infants’ appearance is their transformation into round, plump babies by the middle of the first year. This early rise in “baby fat,” which peaks at about 9 months, helps the infant maintain a constant body temperature. In the second year, most toddlers slim down, a trend that continues into middle childhood (Fomon & Nelson, 2002). In contrast, muscle tissue increases very slowly during infancy and will not reach a peak until adolescence. Babies are not very muscular; their strength and physical coordination are limited.
Figure 5.1 Body growth during the first two years. These photos depict the dramatic changes in body size and proportions during infancy and toddlerhood in two children—a boy, Shanwel, and a girl, Mai. In the first year, the head is quite large in proportion to the rest of the body, and height and weight gain are especially rapid. During the second year, the lower portion of the body catches up. Notice how both children added “baby fat” in the early months of life and then slimmed down, a trend that continues into middle childhood.
ALL PHOTOS © LAURA DWIGHT PHOTOGRAPHY
5.1.2 Changes in Body Proportions
As the child’s overall size increases, parts of the body grow at different rates. Two growth patterns describe these changes. The first is the cephalocaudal trend—from the Latin for “head to tail.” During the prenatal period, the head develops more rapidly than the lower part of the body. At birth, the head takes up one-fourth of total body length, the legs only one-third. Notice how, in Figure 5.1, the lower portion of the body catches up. By age 2, the head accounts for only one-fifth and the legs for nearly one-half of total body length.
In the second pattern, the proximodistal trend, growth proceeds, literally, from “near to far”—from the center of the body outward. In the prenatal period, the head, chest, and trunk grow first; then the arms and legs; and finally the hands and feet. During infancy and childhood, the arms and legs continue to grow somewhat ahead of the hands and feet.
5.1.3 Individual and Group Differences
In infancy, girls are slightly shorter and lighter than boys, with a higher ratio of fat to muscle. These small sex differences persist throughout early and middle childhood and are greatly magnified at adolescence. Ethnic differences in body size are apparent as well. Grace was below the growth norms (height and weight averages for children her age). Early malnutrition contributed, but even after substantial catch-up, Grace—as is typical for Asian children—remained below North American norms. In contrast, Timmy is slightly above average in size, as African-American children tend to be (Bogin, 2001).
Children of the same age differ in rate of physical growth; some make faster progress toward mature body size than others. But current body size is not enough to tell us how quickly a child’s physical growth is moving along. Although Timmy is larger and heavier than Caitlin and Grace, he is not physically more mature. In a moment, you will see why.
The best estimate of a child’s physical maturity is skeletal age, a measure of bone development. It is determined by X-raying the long bones of the body to see the extent to which soft, pliable cartilage has hardened into bone, a gradual process that is completed in adolescence. When skeletal ages are examined, African-American children tend to be slightly ahead of European-American and Hispanic children of the same chronological age. And girls are considerably ahead of boys—the reason Timmy’s skeletal age lags behind that of Caitlin and Grace. At birth, the sexes differ by about 4 to 6 weeks, a gap that widens over infancy and childhood (McCormack et al., 2017; Tanner, Healy, & Cameron, 2001). Girls are advanced in development of other organs as well. This greater physical maturity may contribute to girls’ greater resistance to harmful environmental influences. As noted in Chapter 2, girls experience fewer developmental problems than boys and have lower infant and childhood mortality rates.
5.2 BRAIN DEVELOPMENT
5.2a Describe brain development during infancy and toddlerhood, current methods of measuring brain functioning, and appropriate stimulation to support the brain’s potential.
5.2b Explain how the organization of sleep and wakefulness changes over the first two years.
At birth, the brain is nearer to its adult size than any other physical structure, and it continues to develop at an astounding pace throughout infancy and toddlerhood. We can best understand brain growth by looking at it from two vantage points: (1) the microscopic level of individual brain cells and (2) the larger level of the cerebral cortex, the most complex brain structure and the one responsible for the highly developed intelligence of our species.
5.2.1 Development of Neurons
The typical adult human brain has 100 to 200 billion neurons, or nerve cells, that store and transmit information, many of which have thousands of direct connections with other neurons. Unlike other body cells, neurons are not tightly packed together. Between them are tiny gaps, or synapses, where fibers from different neurons come close together but do not touch (see Figure 5.2). Neurons send messages to one another by releasing chemicals called neurotransmitters, which cross synapses.
The basic story of brain growth concerns how neurons develop and form this elaborate communication system. Figure 5.3 summarizes major milestones of brain development. In the prenatal period, neurons are produced in the embryo’s primitive neural tube. From there, they migrate to form the major parts of the brain (see page 94 in Chapter 3). Once neurons are in place, they differentiate, establishing their unique functions by extending their fibers to form synaptic connections with neighboring cells. During the first two years, neural fibers and synapses increase at an astounding pace (Budday, Steinmann, & Kuhl, 2015; Moore, Persaud, & Torchia, 2016). A surprising aspect of brain growth is programmed cell death, which makes space for these connective structures: As synapses form, many surrounding neurons die—40 to 60 percent, depending on the brain region (Jabès & Nelson, 2014). Fortunately, during the prenatal period, the neural tube produces far more neurons than the brain will ever need.
As neurons form connections, stimulation becomes vital to their survival. Neurons that are stimulated by input from the surrounding environment continue to establish new synapses, forming increasingly elaborate systems of communication that support more complex abilities. At first, stimulation results in a massive overabundance of synapses, many of which serve identical functions, thereby ensuring that the child will acquire the motor, cognitive, and social skills that our species needs to survive. Neurons that are seldom stimulated soon lose their synapses in a process called synaptic pruning that returns neurons not needed at the moment to an uncommitted state so they can support future development. At the same time, pruning allows for rearrangement and strengthening of remaining synapses, which fine-tunes neural circuitry and is essential for effective information processing. In all, about 50 percent of synapses are pruned during childhood and adolescence (Jiang & Nardelli, 2016). For this process to advance, appropriate stimulation of the child’s brain is vital during periods in which the formation of synapses is at its peak.
Figure 5.2 Neurons and their connective fibers. This photograph of several neurons, taken with the aid of a powerful microscope, shows the elaborate synaptic connections that form with neighboring cells.
© CNRI/SCIENCE SOURCE
Figure 5.3 Major milestones of brain development. Formation of synapses is rapid during the first two years, especially in the auditory, visual, and language areas of the cerebral cortex. The prefrontal cortex, responsible for complex thought (see page 159), undergoes more extended synaptic growth. In each area, overproduction of synapses is followed by synaptic pruning. The prefrontal cortex is among the last regions to attain adult levels of synaptic connections—in mid- to late adolescence. Myelination occurs at a dramatic pace during the first two years, more slowly through childhood, followed by an acceleration at adolescence. The multiple yellow lines indicate that the timing of myelination varies among different brain areas. For example, neural fibers myelinate over a longer period in the language areas, and especially in the prefrontal cortex, than in the auditory and visual areas. (Based on Thompson & Nelson, 2001.)
If few neurons are produced after the prenatal period, what causes the extraordinary increase in brain size during the first two years? About half the brain’s volume is made up of glial cells, which are responsible for myelination, the coating of neural fibers with an insulating fatty sheath (called myelin) that improves the efficiency of message transfer. Certain types of glial cells also participate directly in neural communication, by picking up and passing on neuronal signals and releasing neurotransmitters. Glial cells multiply rapidly from the fourth month of pregnancy through the second year of life—a process that continues at a slower pace through middle childhood and accelerates again in adolescence. Gains in neural fibers and myelination account for the overall increase in size of the brain, from nearly 30 percent of its adult weight at birth to 70 percent by age 2 (Budday, Steinmann, & Kuhl, 2015; Terni, López-Murcia, & Llobet, 2017). Growth is especially rapid during the first year, when the brain more than doubles in size.
Brain development can be compared to molding a “living sculpture.” First, neurons and synapses are overproduced. Then, cell death and synaptic pruning sculpt away excess building material to form the mature brain—a process jointly influenced by genetically programmed events and the child’s experiences. The resulting “sculpture” is a set of interconnected regions, each with specific functions—much like countries on a globe that communicate with one another (Johnston et al., 2001). This “geography” of the brain permits researchers to study its organization and the activity of its regions using neurobiological techniques.
Figure 5.4 Electroencephalogram (EEG) using the geodesic sensor net (GSN). Interconnected electrodes embedded in the head cap record electrical brain-wave activity in the cerebral cortex.
OLI SCARFF/GETTY IMAGES
5.2.2 Measures of Brain Functioning
Table 5.1 describes major measures of brain functioning. Among these methods, the two most frequently used detect changes in electrical activity in the cerebral cortex. In an electroencephalogram (EEG), researchers examine brain-wave patterns for stability and organization—signs of mature functioning of the cortex (see Figure 5.4). As a child processes a particular stimulus, event-related potentials (ERPs) can detect the general location of brain-wave activity—a technique often used to study preverbal infants’ responsiveness to various stimuli, the impact of experience on specialization of specific regions of the cortex, and atypical brain functioning in children at risk for learning and emotional problems (DeBoer, Scott, & Nelson, 2007; Gunnar & de Haan, 2009).
Table 5.1 Measuring Brain Functioning
Method
Description
Electroencephalogram (EEG)
Electrodes embedded in a head cap record electrical brain-wave activity in the brain’s outer layers—the cerebral cortex. Researchers use an advanced tool called a geodesic sensor net (GSN) to hold interconnected electrodes (up to 128 for infants and 256 for children and adults) in place through a cap that adjusts to each person’s head shape, yielding high-quality brain-wave detection.
Event-related potentials (ERPs)
Using the EEG, the frequency and amplitude of brain waves in response to particular stimuli (such as a picture, music, or speech) are recorded in multiple areas of the cerebral cortex. Enables identification of general regions of stimulus-induced activity.
Functional magnetic resonance imaging (fMRI)
While the child lies inside a tunnel-shaped apparatus that creates a magnetic field, a scanner magnetically detects increased blood flow and oxygen metabolism in areas of the brain as the individual processes particular stimuli. The scanner typically records images every 1 to 4 seconds; these are combined into a computerized moving picture of activity anywhere in the brain (not just its outer layers). Not appropriate for children younger than age 5 to 6, who cannot remain still during testing.
Positron emission tomography (PET)
After injection or inhalation of a radioactive substance, the person lies on an apparatus with a scanner that emits fine streams of X-rays, which detect increased blood flow and oxygen metabolism in areas of the brain as the person processes particular stimuli. As with fMRI, the result is a computerized image of activity anywhere in the brain. Also like fMRI, not appropriate for children younger than age 5 to 6.
Near-infrared spectroscopy (NIRS)
Using thin, flexible optical fibers attached to the scalp through a head cap, infrared (invisible) light is beamed at the brain; its absorption by areas of the cerebral cortex varies with changes in blood flow and oxygen metabolism as the individual processes particular stimuli. The result is a computerized moving picture of active areas in the cerebral cortex. Unlike fMRI and PET, NIRS is appropriate for infants and young children, who can move within limited range during testing.
Figure 5.5 Functional magnetic resonance imaging (fMRI) and near infrared spectroscopy (NIRS). (a) This 6-year-old is part of a study that uses fMRI to find out how his brain processes light and motion. (b) The fMRI image shows which areas of the child’s brain are active while he views changing visual stimuli. (c) Here, NIRS is used to investigate a 2-month-old’s response to a visual stimulus. During testing, the baby can move freely within a limited range. (Photo (c) from G. Taga, K. Asakawa, A. Maki, Y. Konishi, & H. Koisumi, 2003, “Brain Imaging in Awake Infants by Near-Infrared Optical Topography,” Proceedings of the National Academy of Sciences, 100, p. 10723. Reprinted by permission.)
Neuroimaging techniques, which yield detailed, three-dimensional computerized pictures of the entire brain and its active areas, provide the most precise information about which brain regions are specialized for certain capacities and about abnormalities in brain functioning. In positron emission tomography (PET), the child must lie quietly on a scanner bed, and in functional magnetic resonance imaging (f MRI), inside a tunnel-like apparatus. But unlike PET, fMRI does not depend on X-ray photography, which requires injection of a radioactive substance. Rather, when a child is exposed to a stimulus, fMRI detects changes in blood flow and oxygen metabolism throughout the brain magnetically, yielding a colorful, moving picture of parts of the brain used to perform a given activity (see Figure 5.5a and b).
Because PET and fMRI require that the participant lie as motionless as possible for an extended time, they are not suitable for infants and young children. A neuroimaging technique that works well in infancy and early childhood is near infrared spectroscopy (NIRS) (refer again to Table 5.1). Because the apparatus consists only of thin, flexible optical fibers attached to the scalp using a head cap, a baby can sit on the parent’s lap and move during testing—as Figure 5.5c illustrates. But unlike PET and fMRI, which map activity changes throughout the brain, NIRS examines only the functioning of the cerebral cortex.
The measures just reviewed are powerful tools for uncovering relationships between the brain and psychological development. But like all research methods, they have limitations. Even though a stimulus produces a consistent pattern of brain activity, investigators cannot be certain that an individual has processed it in a certain way (Kagan, 2013b). And a researcher who takes a change in brain activity as an indicator of information processing must make sure that the change was not due instead to hunger, boredom, fatigue, or body movements. Consequently, other methods—both observations and self-reports—must be combined with brain-wave and imaging findings to clarify their meaning. Now let’s turn to the developing organization of the cerebral cortex.
5.2.3 Development of the Cerebral Cortex
The cerebral cortex, resembling half a shelled walnut, surrounds the rest of the brain. It is the largest brain structure—accounting for 85 percent of the brain’s weight and containing the greatest number of neurons and synapses. Each of the 20 billion neurons located in the cerebral cortex has, on average, 7,000 synaptic connections, yielding more than 23,000 miles of myelinated neural fibers (Budday, Steinman, & Kuhl, 2015). Because the cerebral cortex is the last part of the brain to stop growing, it is sensitive to environmental influences for a much longer period than any other part of the brain.
Regions of the Cerebral Cortex
Figure 5.6 shows specific functions of regions of the cerebral cortex, such as receiving information from the senses, instructing the body to move, and thinking. The order in which cortical regions develop corresponds to the order in which various capacities emerge in the infant and growing child. For example, a burst of synaptic growth occurs in the auditory and visual cortexes and in areas responsible for body movement over the first year—a period of dramatic gains in auditory and visual perception and mastery of motor skills (Gilmore et al., 2012). Language areas are especially active from late infancy through the preschool years, when language development flourishes (Pujol et al., 2006).
The cortical regions with the most extended period of development are the frontal lobes. The prefrontal cortex, lying in front of areas controlling body movement, is responsible for complex thought—in particular, consciousness and various “executive” processes, including inhibition of impulses; integration of information; self-regulation of cognition, emotion, and behavior; and memory, reasoning, planning, and problem-solving strategies. From age 2 months on, the prefrontal cortex functions more effectively. But it undergoes especially rapid myelination and formation and pruning of synapses during the preschool and school years, followed by another period of accelerated growth in adolescence, when it reaches an adult level of synaptic connections (Jabès & Nelson, 2014; Jiang & Nardelli, 2016).
Figure 5.6 The left side of the human brain, showing the cerebral cortex. The cortex is divided into different lobes, each of which contains a variety of regions with specific functions. Some major regions are labeled here.
Lateralization and Plasticity of the Cerebral Cortex
The cerebral cortex has two hemispheres, or sides, that differ in their motor, cognitive, and emotional functions. Some tasks are done mostly by the left hemisphere, others by the right—specialization of the hemispheres is called lateralization. For example, each hemisphere receives sensory information from the side of the body opposite to it and controls only that side (vision is a complicated exception). For most of us, the left hemisphere is largely responsible for verbal abilities (such as spoken and written language) and positive emotion (such as joy). The right hemisphere handles spatial abilities (judging distances, reading maps, and recognizing geometric shapes) and negative emotion (such as distress) (Banish & Heller, 1998; Nelson & Bosquet, 2000).
Handedness is the most obvious reflection of cerebral lateralization in humans. In longitudinal research on several hundred ethnically diverse U.S. infants, hand preference in reaching for objects was evident for about half between ages 6 and 14 months. An additional one-fourth began to show a hand preference over the second year; the rest as yet showed no preference (Campbell, Marcinowski, & Michel, 2018; Michel et al., 2014). Like adults, most infants who displayed a hand preference preferred the right hand, which is controlled by the left hemisphere. Nevertheless, numerous studies comparing identical and fraternal twins reveal the heritability of left-handedness to be weak (Ooki, 2014; Vuoksimaa et al., 2009). Although many hypotheses about early environmental influences on handedness exist, none is consistently supported by research.
Handedness, ho
Collepals.com Plagiarism Free Papers
Are you looking for custom essay writing service or even dissertation writing services? Just request for our write my paper service, and we'll match you with the best essay writer in your subject! With an exceptional team of professional academic experts in a wide range of subjects, we can guarantee you an unrivaled quality of custom-written papers.
Get ZERO PLAGIARISM, HUMAN WRITTEN ESSAYS
Why Hire Collepals.com writers to do your paper?
Quality- We are experienced and have access to ample research materials.
We write plagiarism Free Content
Confidential- We never share or sell your personal information to third parties.
Support-Chat with us today! We are always waiting to answer all your questions.
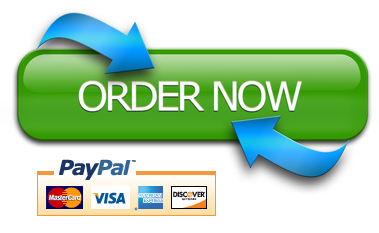