Week 5 Article Critique Use the Campbellsville University Library databases to do research on peer-reviewed journal articles on the topic of Current and Emer
Week 5 Article Critique
Use the Campbellsville University Library databases to do research on peer-reviewed journal articles on the topic of Current and Emerging Technology (do not use Google or Wikipedia). Choose an article that includes all parts listed in the Article Critique Rubric located on the Moodle course page. Download the file in the attachment below to type in your responses, then upload the completed file.*After downloading the word document below, type your responses directly into the word file.
*Students should type directly into the chart below.
ARTICLE CRITIQUE
Parts of Article Critique |
Student Responses |
Author(s) First and Last Name |
|
Article Title |
|
Publication Date: Year (within last 10 years) |
|
Journal Name |
|
Journal Volume |
|
Journal Number |
|
Journal Pages (range, ex. 1-10) |
|
Article Abstract: highlight and copy the exact abstract from the article chosen and paste the abstract here |
|
Takeaway: In a bulleted list, write complete sentences about three things you have learned from the article. *The takeaway should be written in your own words with no similarity. |
· · · |
Bluestein, H. B., Rauber, R. M., Burgess, D. W., Albrecht, B., Ellis, S. M., Richardson, Y. P., Jorgensen, D. P., Frasier, S. J., Chilson, P., Palmer, R. D., Yuter, S. E., Lee, W.-C., Dowell, D. C., Smith, P. L., Markowski, P. M., Friedrich, K., & Weckwerth, T. M. (2014). RADAR IN ATMOSPHERIC SCIENCES AND RELATED RESEARCH : Current Systems, Emerging Technology, and Future Needs. Bulletin of the American Meteorological Society, 95(12), 1850–1861.
,
RADAR IN ATMOSPHERIC SCIENCES AND RELATED RESEARCH Current Systems, Emerging Technology, and Future Needs
by Howard b. bluestein, robert M. rauber, donald w. burgess, bruce albrecHt, scott M. ellis, yvette P. ricHardson, david P. Jorgensen, stePHen J. Frasier, PHilliP cHilson, robert d. PalMer, sandra e. yuter, wen-cHau lee, david c. dowell, Paul l. sMitH, Paul M. Markowski, katJa FriedricH, and taMMy M. weckwertH
Emerging radar technologies best suited to addressing key scientific questions and future problems are identified and discussed.
T o help its Lower Atmosphere Observing Facilities (LAOF)
program provide researchers with instrumentation and
platforms, the National Science Foundation (NSF) con-
vened a community workshop1 with the purpose of defining
the problems of the next generation that will require radar
technologies and to determine the suite of radars best suited
to solving these problems. The workshop addressed a subset
of the instruments2 mentioned by the National Research
Council (2009) as being or eventually becoming essential
operational facilities. Climate scientists have also recom-
mended similar reviews of instrumentation, in part because
“we may . . . need easily deployable short-term observational
technologies to monitor potential abrupt changes or impor-
tant regional trends” (National Research Council 2010).
Based on presentations and discussions at the NSF work-
shop (Figs. 1 and 2), we considered the following questions:
1 The workshop was held at the NCAR in Boulder, Colorado, from 27 to 29 November 2012. There were over 120 participants who repre- sented six countries (United States including Hawaii, Canada, France, Germany, Japan, and Taiwan) and three continents. Additional par- ticipants attended by web conferencing. More information about the workshop including presentations and posters may be found online (at www.joss.ucar.edu/nsf_radar_wksp_nov12/).
2 Spaceborne radars, which would require collaboration among NASA, NOAA, and NSF, were not considered during the workshop.
A rapid-scan, X-band, polarimetric, Doppler radar (RaXPol) probing a supercell in southwestern Oklahoma on 23 May 2011. (Courtesy of H. Bluestein.)
1) What current radar technologies are considered critical to answering the key current and emerging scientific questions? What are the strengths and weaknesses of those technologies as they are cur- rently implemented?
2) What emerging radar technologies would be most helpful in answering the key scientif ic questions?
3) W hat gaps, if a ny, ex ist in rada r obser v ing technologies? (A “gap” can mean the absence of a critical technology or a lack of access by the general research community to an existing technology.)
While we address these questions here, an online supplement (available online at http://dx.doi.org/10.1175 /BAMS-D-13-00079.2) reviews in more depth the radar technolog y currently in use (see Tables 1–3 for sur veys of current ground-based mobile, airborne, and deployable radars, respectively) and provides more information about the work- shop itself.
E M E R G I N G R E S E A R C H FRONTIERS. Future research3 with radar will involve multiplat- form, multimodel investigations. There will be four major research themes involving radar.
Extreme weather. Studies of tropical- cyclone genesis including spatial structure, rapid intensification, and
evolution during landfall, convective weather systems, and winter weather systems will all require radar. It was proposed that this should include a rapid-response capability to facilitate study of extreme weather events that are predictable days in advance.
Hydrology and water resources. Studies of tropical– extratropical transition, f looding from landfalling tropical systems, midlatitude convective systems, atmospheric rivers, the interaction of weather systems with orography and topography, weather modifica- tion for precipitation enhancement, and mapping the water vapor field for numerical weather prediction
3 This section is based on a presentation by Robert Rauber at the workshop.
Fig. 1. Small discussion group (from left to right: John Williams, Bob Palmer, Jim Wilson, and Phil Chilson) during the radar analysis and software session. (Courtesy of H. Bluestein.)
AFFILIATIONS: bluestein—School of Meteorology, University of Oklahoma, Norman, Oklahoma; cHilson and PalMer—School of Meteorology, and Advanced Radar Research Center, University of Oklahoma, Norman, Oklahoma; rauber—University of Illinois at Urbana–Champaign, Urbana, Illinois; burgess—Cooperative Institute for Mesoscale Meteorological Studies, Norman, Oklahoma; Jorgensen—National Oceanic and Atmospheric Administration/ National Severe Storms Laboratory, Norman, Oklahoma; albrecHt— University of Miami, Miami, Florida; ellis, lee, and weckwertH— National Center for Atmospheric Research, Boulder, Colorado; ricHardson and Markowski—The Pennsylvania State University, University Park, Pennsylvania; Frasier—Microwave Remote Sensing Laboratory, University of Massachusetts Amherst, Amherst, Massachusetts; yuter—North Carolina State University, Raleigh, North Carolina; dowell—National Oceanic and Atmospheric
Administration/Earth System Research Laboratory, Boulder, Colo- rado; sMitH—South Dakota School of Mines and Technology, Rapid City, South Dakota; FriedricH—University of Colorado, Boulder, Colorado CORRESPONDING AUTHOR : Howard B. Bluestein, School of Meteorology, University of Oklahoma, 120 David L. Boren Blvd., Suite 5900, Norman, OK 73072 E-mail: [email protected]
The abstract for this article can be found in this issue, following the table of contents. DOI:10.1175/BAMS-D-13-00079.1
A supplement to this article is available online (10.1175/BAMS-D-13-00079.2)
In final form 8 January 2014 ©2014 American Meteorological Society
1852 DECEMBER 2014|
and estimating precipitation rates will also all require radar.
Global and regional climate and climate change. Radar will be needed to study, for example, tropical cloud systems, oceanic tropical convection and its
impact on climate, the effects of aerosols on cloud microphysics, and global and regional orographic precipitation as a source of water supply. Dr. Rauber suggests that during the next two decades global climate change impacts are likely to drive research more and more.
I n t e g r a t i o n o f r a d a r d a t a w i t h numerical models. This particularly applies to developing high-resolu- tion regional and global models that make use of assimilated radar data.
To address these scientific prob- lems , rad a rs w i l l be needed on diverse platforms including ships, aircraft, and stationary and mobile ground-based platforms. Some of the latter platforms may be quasi mobile: able to move the radar to a f ixed location for an extended period. Other radars will be perma- nently installed at fixed locations and may be part of a network of either high-powered, widely spaced r ad a rs or low-powered , closely
Fig. 2. Participants (Dave Jorgensen on the left; unidentified on the right) checking lists of priorities posted on the walls (gallery walk) during radar analysis and software session. (Courtesy of H. Bluestein.)
Table 1. Ground-based mobile radars survey.
Name Band Beamwidth Polarization Scan rate Owner
Shared Mobile Atmospheric Research and Teaching Radar (SMART-R)
C 1.5° 1 dual, 1 single
33° s-1 University of Oklahoma (OU)/
Texas Tech
Seminole Hurricane Hunter C — single 10° s-1 Florida State University (FSU)
Doppler on Wheels (DOW) X 0.9° 2 dual 50° s-1 Center for Severe Weather
Research (CSWR)
NOAA X-band, dual-Polarized radar (NOXP)
X 1° dual 30° s-1 NOAA
UMass dual-polarized X-band mobile Doppler radar (X-Pol)
X 1.25° dual 20° s-1 UMass
Mobile Alabama X-Band (MAX) X 1° dual 18° s-1 University of Alabama in
Huntsville (UAH)
Texas Tech Ka-band (TTUKa) Ka 0.33° 2 single 20° s-1 Texas Tech
UMass W-band W 0.18° dual 5° s-1 UMass
Rapid DOW X 1° single 50° s-1 × 6 beams CSWR
Rapid-scanning, X-band, polarimetric mobile radar (RaXPol)
X 1° dual 180° s-1 OU
Mobile Weather Radar, 2005 X-band, Phased Array (MWR-05XP)
X 1.8° × 2° single 180° s-1
up to 55°
Naval Postgraduate School (NPS) Center for Interdis- ciplinary Remotely-Piloted Aircraft Studies (CIRPAS)
UMass X-band phase-tilt array X 2° × 3.5° dual 90° s-1 UMass
AIR X 1° × 25° single 40° s-1 OU
PX-1000 X 1.9° dual 50° s-1 OU
1853DECEMBER 2014AMERICAN METEOROLOGICAL SOCIETY |
spaced radars. There will also be a diversity of scan- ning strategies, ranging from traditional scanning radars [for sur veillance, range–height indicator (RHI), and sector scans] to rapid-scan radars and vertically pointing profilers, as well as airborne scan strategies and airborne profilers. There will also be a need for diversity of wavelengths and polarization configurations. Typical bands (wavelengths) used range over the S (~10 cm), C (~5 cm), X (~3 cm), Ka/Ku (~1 cm), and W (~3 mm) bands and will vary according to the platform used. Radars will usually const it ute just one component of mu lt isensor,
multiplatform, and multimodel investigations of meteorological phenomena.
EMERGING TECHNOLOGIES. Emerging tech- nologies in meteorological radar4 include solid-state transmitters with pulse compression, polarimetric phased-array radar, imaging radar (or “ubiquitous radar”), and “adaptive collaborative” radar net- works such as the Collaborative Adaptive Sensing of the Atmosphere (CASA; McLaughlin et al. 2009).
Table 2. Airborne radars survey.
Radar name Hurricane surveillance NOAA P-3 ELDORA
Wyoming Cloud Radar (WCR)
Owner NOAA [Environmental
Science Services Administration (ESSA)]
NOAA NSF/NCAR University of Wyoming
Platform DC-6 P-3 Orion 4-engine
turboprop Electra, NRL P-3 King-Air, C-130
Scanning RHI scan tied to drift angle RHI–fore–aft ±20° normal to heading
RHI–fore–aft ±20° normal to heading
Vertical or horizontal dual beam
Radar type Noncoherent Doppler Doppler Doppler, dual pol
Frequency X band X band X band W band
Years ~1964–75 1977–present 1992–2012 1995–present
Radar name
ER-2 Doppler Radar (EDOP)
Cloud Radar System (CRS)
High-Altitude Imaging Rain and Wind Profiler
(HIWRAP) ER-2 X-band Radar
(EXRad)
Owner National Aeronautics
and Space Administration (NASA)
NASA NASA NASA
Platform ER-2 ER-2 WB-57, Global Hawk ER-2
Scanning Dual beam
(fixed, nadir and +35°) Nadir Nadir conical, dual beam
Dual beam: conical or cross-track scan about nadir;
fixed nadir
Radar type Doppler, dual pol (LDR) Doppler, dual pol (LDR) Doppler Doppler
Frequency (band)
X W K u , K
a X
Years 1993–present 2002–present 2010–present 2010–present
4 Reviewed by R. Palmer at the workshop.
1854 DECEMBER 2014|
Future technologies include “digital at every element” phased-array radar, passive radar, multimission networks, ultra low-cost dense networks, and spec- trum sharing.
Solid-state pulse-compression transmitters, which are commercially available, make use of gallium arsenic or gallium nitride technologies. They are low cost, low weight, and low power (so they need longer pulses to achieve high sensitivity) but enable active phased-array and ultra low-cost radars, such as those to be placed on airborne platforms or in large, densely spaced networks. Challenges to their full
implementation include suppressing range sidelobe effects and correcting for blind range (Cheong et al. 2013). For conventional pulsed radars, the range resolution degrades as the pulse duration increases. However, using frequency modulated waveforms the range resolution is decoupled from pulse duration and instead is inversely proportional to bandwidth. Unfortunately, range sidelobe contamination and blind range challenges (at close range) are introduced; the nature of the nonlinear modulation must be optimized to reduce the former. Short-pulse blind range-filling techniques are available, but they create
Table 2. Continued.
Radar name
Super Polarimetric Ice-crystal Detection and Explication Radar
(SPIDER)
Environmental Canada Cloud Profiling Radar
(EC CPR) Radar System Airborne
(RASTA)
National Research Council (NRC) Airborne W and
X-band Polarimetric Doppler Radar (NAWX)
Owner Japan Canada France Canada
Platform Gulfstream-II Convair-580 Falcon 20, ATR-42 Convair 580
Scanning -40° to +95° scan across flight direction
Fixed zenith and nadir looking
3 down beams, 2 up beams; ±15° sector
Vertical and side looking
Radar type Doppler, dual pol Noncoherent Doppler Doppler, dual pol
Frequency (band)
W K a
W X, W
Years 1998–present 1999–present 2000–present 2006–present
Radar name
G-IV Tail Doppler Radar
High-Performance Instrumented
Airborne Platform for Environmental
Research (HIAPER) Cloud Radar (HCR)
Airborne Cloud Radar (ACR)
Imaging Wind and Rain Profiler (IWRAP)
Owner NOAA NSF NCAR NASA UMass
Platform NOAA G-IV NSF G-V NASA P-3, DC-8 NOAA P-3
Scanning RHI-Fore-Aft ±20° normal to heading
RHI Nadir Conical scan about nadir, quad beam (30°, 35°, 40°, and 50°)
Radar type Doppler Doppler, dual pol Doppler dual pol Doppler dual pol
Frequency (band)
X W W C, K u
Years 2010 2013–present 1995–present 2002–present
1855DECEMBER 2014AMERICAN METEOROLOGICAL SOCIETY |
an abrupt change in sensitivity at the transition from short to long pulse. Aside from the commercially available pulse compression systems, others are mobile systems at Ka and X bands (Table 2).
T h e m a i n c h a l l e n g e s t o i n c l u d i n g d u a l – polarimetric capabilities on phased-array antennas are that horizontal–vertical orthogonality is not preserved as the phased-array antenna is steered off the principal planes and that the antenna gain and beamwidth change for off-broadside pointing direc- tions. Engineering development efforts are ongoing at several places, including Massachusetts Institute of Technology Lincoln Laboratories (MIT-LL) for an S-band overlapped subarray, BCI/Lockheed Martin for an S-band 12 × 12 element prototype, and the National Center for Atmospheric Research (NCAR) for a C-band airborne system. Meanwhile, actual weather measurements have been made successfully with an X-band phase tilt antenna from collaboration between the University of Massachusetts (UMass) and Raytheon (“phase-tilt radar”; Orzel et al. 2011) and are planned at the University of Ok lahoma (OU) for an S-band cylindrical antenna [Cylindrical Polarimetric Phased Array Radar (CPPAR); Zhang et al. 2011] for the National Severe Storms Laboratory (NSSL) Multi-Function Phased Array Radar (MPAR) program (Zrnić et al. 2007; Weber et al. 2007). The solution to the polarization orthogonality problem for both of these designs is to stay on the principal planes while scanning. The former operates at X band, steers electronically in azimuth and mechani- cally in elevation, has a 2.5°–3.5° half-power beam- width, is low powered, and makes use of nonlinear frequency modulated pulse compression; the latter operates at S band, is fully electronically steered using commutating beams around the cylinder (but
is frequency steered in elevation for cost reduction), is high powered, and has 4.5° half-power beamwidth.
An alternative to (analog) phased-array technolo- gy for rapid scanning is the imaging-radar technique, in which a relatively broad region is illuminated by the transmitted beam. Digital beamforming (by which the phase and amplitude of the signal received at each antenna element are altered digitally at each separate receiver for each antenna element, rather than through separate phase delays for each element, but with just one receiver for all antenna elements) allows for simultaneous measurements with an infi- nite number (not all independent) of receiving beams each of a given half-power beamwidth: for example, of 1°. The first radar to make use of this technique is the Atmospheric Imaging Radar (AIR) at OU (Isom et al. 2013), in which an elliptical transmitted beam, narrow in azimuth but wide in elevation, illuminates the volume. This radar operates at X band and pro- duces instantaneous RHIs but is scanned mechani- cally in azimuth. In the future, it will be possible to scan both in elevation and in azimuth through the imaging-radar technique using digital beamforming in two dimensions, not just one. A major advantage is that ground clutter is rejected via adaptive array processing (e.g., spatial filtering). This type of clutter filtering can also reject moving targets, such as air- craft and wind turbines. To date, data from the AIR have been processed by the Fourier method and the robust Capon method, each of which has strengths and weaknesses. So far the AIR has been used to probe tornadoes.
The Toshiba Corporation has designed an X- band phased-array radar system that makes use of both an active phased-array antenna and digital beamforming. The 1° half-power beamwidth antenna
Table 3. Deployable-radars survey.
Large systems Medium sized Small Zenith pointing
NCAR S-Pol ARM X/Ka University of Iowa X-Pol ARM Ka-band zenith radar (KaZR)
[Millimeter Wave Cloud Radar (MMCR)]
CSU–CHILL ARM Ka/W OU PX-1000 W-band ARM Cloud Radar (WACR)
NASA N-Pol NASA D3R (Ku/Ka) CASA Integrated Project 1
(IP-1) Earth System Research Laboratory
(ESRL) W band
UMass Advanced Multi-Frequency Radar (AMFR) (Ku/Ka/W)
ESRL S band
ARM C band
MIT-LL C band
Long range: precipitation, clear air
Short–long range: clouds, precipitation
Short range: precipitation, networks
Profiling: clouds, precipitation, microphysics
1856 DECEMBER 2014|
scans mechanically in azimuth and electronically in elevation. Low powered and making use of pulse com- pression, it is installed at Osaka University in Japan.
While CASA is a concept in a networked radar system that has been tested in the United States (McLaughlin et al. 2009), in Japan there is another closely spaced network of X-band, solid-state, low- powered, lightweight radars called WITH, built by Weathernews, Inc. These are retrofitted radars having a half-power beamwidth of 6° and are highly mobile (can be easily towed by a car, put on top of buildings, etc.).
In the future, digital-at-every-element phased- array antennas will become available. The real-time computational load will be tremendous, but the degrees of freedom for adaptive beamforming, etc., will be huge. An additional major challenge with such radars will be calibration.
Also in the future, it may be possible for passive radar networks to be set up using “transmitters of opportunity” such as those from television stations, FM radio stations, and cellular phone networks. Examples of such networks include Bistatic Network (BiNet) (Wurman et al. 1993; Friedrich and Hagen 20 04), using Weat her Sur vei l la nce Rada r-1988 Doppler (WSR-88D) radars, and the U.S. Department of Defense Integrated Sensor Is the Structure (ISIS), using FM radio transmitters.
Multimission radar networks that can be used for aircraft surveillance, weather observations, and communications may be built with the aim of savings resulting from decreasing the overall number of radars used. Ultra low-cost dense networks making use of solid-state transmitters with pulse compression may be implemented. However, the weather-radar community will face competition from the wireless communication industry, which wants to use some of the weather-radar frequency bands. Some coopera- tion and coordination of functions will be required. Possible solutions include passive radar networks, adaptive phased-array radar systems (which have tremendous advantages for beam agility and coor- dination, and interference suppression), and other forms of multimission networks.
RECOMMENDATIONS. To address high-priority research topics, specific radars on specific platforms will be required. We summarize the community’s opinion (as it emerged from workshop discussions) of both the specific types of radars and the platforms needed and also the opportunities for radar support of high-priority research. The following findings and rec- ommendations are not ordered according to priority.
Polarimetric radars are needed to study pre- cipitation processes, including those associated with f looding in general and f lash f looding specifically; to study cloud processes, especially those associated with mixed-phase clouds; and to study insect and bird behavior and migration. Measurements are espe- cially needed to characterize ice microphysics better, perhaps also using polarization modes other than orthogonal linear. Errors related to dual-polarization technology need to be quantified better, particularly in the simultaneous transmit/receive mode. There should be movement toward quantif ying micro- physical processes and hydrometeor distributions in four dimensions. Hydrometeor classification could be taken to a new level with new instrumentation such as the A-10 aircraft (www.cirpas.org/a10spa .html) and techniques such as dual-polarization and multifrequency radar techniques, which are cur- rently underutilized. Polarimetric radar observa- tions could also be used for electrification research. Researchers need to be made aware of the different uses of WSR-88D data and, in particular, for the study of ice microphysics, despite the difficulties in using data when both the horizontal and vertical beams are transmitted simultaneously. Real verification of dual- polarization measurements on large scales is needed.
S-band, Bragg scattering radars are needed to study chemical transport and also to study features in clear air, such as upper-level fronts and jets, low-level jets, and surface boundaries along which convective initiation may occur. Currently CASA radars (and in general low-cost radar networks) lack clear-air data collection capabilities, since monos- tatic X-band radars cannot detect Bragg scattering in clear air. Operational refractivity measurements from the WSR-88D radars need to be developed and exploited so that mesoscale detail in the temperature and moisture fields can be better resolved. The com- munity would like to have access to mobile, S-band radar systems with a 1° half-power beamwidth or less. Soon a transportable (trailer towed) radar will become available from the Naval Postgraduate School (NPS) Center for Interdisciplinary Remotely-Piloted Aircraft Studies (CIRPAS), but with lower spatial resolution.
Airborne Electra Doppler Radar (ELDORA)-like radars are needed to study convection and tropical cyclogenesis over the ocean and convection over land, especially in mountainous or remote areas where ground-based radars cannot be easily deployed (Hildebrand et al. 1994). The existing ELDORA has not been requested much lately because it is difficult to process its data and Naval Research Laboratory
1857DECEMBER 2014AMERICAN METEOROLOGICAL SOCIETY |
(NRL) support is also needed to f ly the P-3. It was suggested that perhaps NSF-sponsored users could piggyback on National Oceanic and Atmospheric Administration (NOAA) experiments. As alternatives to ELDORA, however, the NOAA P-3 radars are dif- ficult to use and expensive and involve a complicated process to obtain; use of them needs to be coordi- nated with other NOAA experiments. Operations of a NOAA P-3 and ELDORA would be less expensive if they could be deployed for short periods of time (e.g., just a few days) rather than for long field campaigns with long waiting periods. NSF needs to establish a mechanism to funnel funds through NOAA so the cost of using a NOAA P-3 falls. There was brief discussion on how to speed up the replacement of ELDORA, since the NOAA P-3 is not available for 6 months out of the year and is very expensive. There is also a need for replacing ELDORA with multiple- waveleng th, dua l-polarization capabilit y. W.-C. Lee noted that EOL at NCAR has been evaluating whether the Airborne Phased-Array Radar (APAR) development (replacement for ELDORA) time can be accelerated with more funding by making it possible to perform some tasks simultaneously rather than sequentially.
There should be support for a diversity of radar platforms at several wavelengths. Estimates of snow, ice, and cloud properties and the retrieval of cloud water and water vapor fields will make use of more multiple-wavelength radars, benefiting a wide range of atmospheric studies. It is difficult to study cloud and precipitation processes owing to their high spatial and temporal variability, so improved resolution is required. The range resolution might be sufficient, but angular resolution depends on the antenna size and the range from the radar; shorter-wavelength radars might yield useful cross-range resolution while maintaining antenna dish size. The use of multiple- wavelength radars might solve help mitigate attenu- ation problems at short wavelengths.
There already is a good mix of truck-mounted mobile radars (including rapid-scan radars) at various wavelengths (C, X, Ka/Ku, and W bands), which need to be maintained. Multiple radars mounted on the same platform are needed to study the microphysics and dynamics of precipitation systems and convec- tive storms. Improvements are needed in instru- ment integration, so that combined measurements of kinematics, thermodynamics, and microphysics at fine spatial and temporal resolution can be made. Multiple-wavelength airborne radars are also needed.
A very high-frequency (VHF) profiling system with Bragg scattering capabilities for boundar y
layer applications might be developed and included in the deployment pool for making temperature and moisture observations; this capability could include three dimensions if a network is developed. Since mov ing many prof ilers is ver y expensive, perhaps a network of deployable profilers could be developed. Wind and thermodynamic observations above the planetary boundary layer are also needed; such prof ilers should be developed. Thermody- namic profiling radars are required also for study- ing mesoscale features in phenomena such as winter storms and for studying convective initiation. Cloud (high frequency) radars are needed to study cloud and precipitation microphysics associated with convec- tive storms, tropical convection, winter storms, and orographic precipitation.
There is a need to have the capability to make radar observations in data-sparse regions, such as near the poles, over the oceans, and in mountainous areas; to do so, we need, in addition to airborne radars such as ELDORA, ship-based radars and perhaps buoy-borne radars as well. Such radar observations are important for climate research related to tropical convection and polar clouds (e.g., for ice microphysics studies). The concepts of how to move facilities to remote areas should be improved. We need to cons
Collepals.com Plagiarism Free Papers
Are you looking for custom essay writing service or even dissertation writing services? Just request for our write my paper service, and we'll match you with the best essay writer in your subject! With an exceptional team of professional academic experts in a wide range of subjects, we can guarantee you an unrivaled quality of custom-written papers.
Get ZERO PLAGIARISM, HUMAN WRITTEN ESSAYS
Why Hire Collepals.com writers to do your paper?
Quality- We are experienced and have access to ample research materials.
We write plagiarism Free Content
Confidential- We never share or sell your personal information to third parties.
Support-Chat with us today! We are always waiting to answer all your questions.
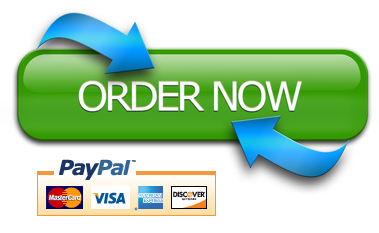