Assessment criteria include, paper format, content literature cited and article choice. In addition to the three articles used f
Assessment criteria include, paper format, content literature cited and article choice. In addition to the three articles used for analysis, supporting references should also be used. A minimum of one unique supporting article is required in the Introduction and Conclusion sections. A student example paper has been uploaded to Blackboard. This paper is well-done and hits almost all the “Meets expectations” criteria in the rubric.
Summary sums up the strengths and weaknesses of each article; compares and contrasts articles to one another summary lacks some details, misses a strength/weakness of one of the articles; occasionally compares and contrasts but mainly lists each articles strengths and weakness separately summary lacks detail, includes either strengths or weaknesses; fails to compare and contrasts articles to one another
Significance establishes practical and theoretical significance of body of work; has your chosen article been cited by others; did your articles spark other researches hypotheses or questions; are there any practical applications; implication (social, political, technological, medical) to the research; cites at least one other supporting reference (unique from introduction) logic not clear to the theoretical significance of the body of work; not thorough in establishing its significance; cites at least one other supporting reference (unique from introduction) no connection to theoretical significance of body of work; fails to cite at least one supporting reference Literature cited Format one journal format chosen and used throughout in bibliography and in-text citations some in-text citations were not in the same format; 1-2 errors in bibliography consistency lacking for in-text citations; bibliography with 3+ formatting errors Subject Chosen articles were all on the same topic; topic was specific enough so that an analysis was possible topics were not consistent or were too broad/general each article was on a separate topic and the topics were without reasonable similarities Citation Each reference was used and cited correctly within the body of the paper; three focal references were analyzed; at least 5 references used references were occasionally cited incorrectly; three focal references were analyzed; 4 total references used two or fewer references were analyzed; no supporting references used Quantity minimum of 5, 1 unique to intro, 1 unique to discussion and 3 critically reviewed missing 1 unique missing 2 unique and/or 1 of critically reviewed.
Host-Microbe Coevolution: Applying Evidence from Model Systems to Complex Marine Invertebrate Holobionts
Paul A. O’Brien,a,b,c Nicole S. Webster,b,c,d David J. Miller,e,f David G. Bournea,b,c
aCollege of Science and Engineering, James Cook University, Townsville, QLD, Australia bAustralian Institute of Marine Science, Townsville, QLD, Australia [email protected], Townsville, QLD, Australia dAustralian Centre for Ecogenomics, University of Queensland, Brisbane, QLD, Australia eARC Centre of Excellence for Coral Reef Studies, James Cook University, Townsville, QLD, Australia fCentre for Tropical Bioinformatics and Molecular Biology, James Cook University, Townsville, QLD, Australia
ABSTRACT Marine invertebrates often host diverse microbial communities, making it difficult to identify important symbionts and to understand how these communi- ties are structured. This complexity has also made it challenging to assign microbial functions and to unravel the myriad of interactions among the microbiota. Here we propose to address these issues by applying evidence from model systems of host- microbe coevolution to complex marine invertebrate microbiomes. Coevolution is the reciprocal adaptation of one lineage in response to another and can occur through the interaction of a host and its beneficial symbiont. A classic indicator of coevolution is codivergence of host and microbe, and evidence of this is found in both corals and sponges. Metabolic collaboration between host and microbe is of- ten linked to codivergence and appears likely in complex holobionts, where micro- bial symbionts can interact with host cells through production and degradation of metabolic compounds. Neutral models are also useful to distinguish selected mi- crobes against a background population consisting predominately of random associ- ates. Enhanced understanding of the interactions between marine invertebrates and their microbial communities is urgently required as coral reefs face unprecedented local and global pressures and as active restoration approaches, including manipula- tion of the microbiome, are proposed to improve the health and tolerance of reef species. On the basis of a detailed review of the literature, we propose three re- search criteria for examining coevolution in marine invertebrates: (i) identifying sto- chastic and deterministic components of the microbiome, (ii) assessing codivergence of host and microbe, and (iii) confirming the intimate association based on shared metabolic function.
KEYWORDS codivergence, coevolution, marine invertebrates, microbiome, phylosymbiosis
Coevolution theory dates back to the 19th century (box 1), and coevolution iscurrently referred to as the reciprocal evolution of one lineage in response to another (1). This definition encompasses a broad range of interactions such as predator- prey, host-symbiont, and host-parasite interactions or interactions among the members of a community of organisms such as a host and its associated microbiome (1, 2). In the case of host-microbe associations, this has produced some of the most remarkable evolutionary outcomes that have shaped life on Earth, such as the eukaryotic cell, multicellularity, and the development of organ systems (3, 4). It is now recognized that microbial associations with a multicellular host represent the rule rather than the
Citation O’Brien PA, Webster NS, Miller DJ, Bourne DG. 2019. Host-microbe coevolution: applying evidence from model systems to complex marine invertebrate holobionts. mBio 10:e02241-18. https://doi.org/10.1128/mBio .02241-18.
Editor Danielle A. Garsin, University of Texas Health Science Center at Houston
Copyright © 2019 O’Brien et al. This is an open-access article distributed under the terms of the Creative Commons Attribution 4.0 International license.
Address correspondence to David G. Bourne, [email protected]
Published 5 February 2019
MINIREVIEW Host-Microbe Biology
crossm
January/February 2019 Volume 10 Issue 1 e02241-18 ® mbio.asm.org 1
D ow
nl oa
de d
fr om
h tt
ps :/
/j ou
rn al
s. as
m .o
rg /j
ou rn
al /m
bi o
on 0
2 F
eb ru
ar y
20 22
b y
24 .1
16 .2
51 .2
22 .
exception (4), but in complex associations of that kind, the extent to which coevolution operates is often unclear.
BOX 1: A BRIEF HISTORY OF COEVOLUTION Charles Darwin once explained the sudden and rapid diversification of flowering
plants as an “abominable mystery,” since it could not be explained by traditional views of evolution alone (5). While his correspondent Gaston de Saporta speculated that a biological interaction between flowering plants and insects might be the cause of the phenomenon, it was not until nearly 100 years later that the concept of coevolution developed. In a pioneering study, Ehrlich and Raven (6) observed that related groups of butterflies were feeding on related groups of plants and specu- lated this was due to a process for which they coined the name “coevolution.” Using butterflies, they argued that plants had evolved mechanisms to overcome predation from herbivores, which in turn had evolved new ways to prey on plants. Decades on, the introduction of phylogenetics has shown that plants evolved in the absence of butterflies, which colonized the diverse group of plants after their chemical defenses were already in place (7). Nevertheless, the theory of coevolution was endorsed, and two important points came to light. First, care must be taken when inferring coevolution from seemingly parallel lines of evolution, and where possible, diver- gence times and common ancestry should be included. Second, coevolution can occur between communities of organisms (“guild” coevolution), as observed in the case of flowering plants, where predation and pollination from a wide variety of insects likely influenced the diversification of angiosperms (8).
Since coevolution can occur across multiple levels of interactions, multiple theories have also developed. The Red Queen theory is based on the concept of antagonistic coevolution and assumes that an adaptation that increases the fitness of one species will come at the cost to the fitness of another (9). This type of coevolution has been most pronounced in host-parasite interactions, where the antagonistic interactions are closely coupled (10). However, coevolutionary patterns may also arise in the case of mutualistic symbioses, which require reciprocal adaptations to the benefit of each partner (11). Mutualistic coevolution is associated with a number of key traits that are discussed further in this review, such as obligate symbiosis, vertical inheritance, and metabolic collaboration. Third, coevolution has also recently been placed in context of the hologenome theory (12), which suggests that the holobiont can act as a unit of selection (but not necessarily as the primary unit) since the combined genomes influence the host phenotype on which selection may operate (13, 14). However, hologenome theory also acknowledges that selection acts on each component of the holobiont individually as well as in combination with other components (including the host). Thus, the entity that is the hologenome may be formed, in part, through coevolution of interacting holobiont compartments, in addition to neutral processes (12).
Given the ubiquitous nature of host-microbe associations and the huge metabolic potential that microorganisms represent, it is not surprising that evidence of host- microbe coevolution is emerging. Model representatives of both simple and complex associations are being used to study coevolution, allowing researchers to look for specific traits, signals, and patterns (1, 15). A well-known model system is the pea-aphid and its endosymbiotic bacteria in the genus Buchnera. This insect has evolved special- ized cells known as bacteriocytes to host its endosymbionts, which in turn synthesize and translocate amino acids that are missing from the diet of the pea aphids (16). Amino acid synthesis occurs through intimate cooperation between host and symbiont, with some pathways missing from the host and some from the symbiont, such that the relationship is obligate to the extent that the one organism cannot survive without the other (17). The human gut microbiome has been extensively studied in complex systems and has been shown to be intimately associated with human health. Gut
Minireview ®
January/February 2019 Volume 10 Issue 1 e02241-18 mbio.asm.org 2
D ow
nl oa
de d
fr om
h tt
ps :/
/j ou
rn al
s. as
m .o
rg /j
ou rn
al /m
bi o
on 0
2 F
eb ru
ar y
20 22
b y
24 .1
16 .2
51 .2
22 .
microbes have been shown to be linked with human behavior and development through metabolic processes, such as microbial regulation of the essential amino acid tryptophan (18, 19). The human microbiome contains around 150-fold more nonre- dundant genes than the human genome (20), and the metabolic capacity of microbes residing in the intestine is believed to have been a driving evolutionary force in the host-microbe coevolution of humans (2). In these examples, as well as many others (21–23), both host and symbiont evolved to maintain and facilitate the symbiosis. Furthermore, phylogenies of host and symbiont in these systems are often mirrored, indicating that host and symbiont are diverging in parallel (16, 24, 25), a phenomenon known as codivergence (26).
In the marine environment, invertebrates can host microbial communities as simple and stable as that of the pea aphid or as complex and dynamic as that of the human gut (Fig. 1). The Hawaiian bobtail squid, for example, maintains an exclusive symbiosis with a single bacterial symbiont which it hosts within a specialized light organ (27). On the other hand, corals host enormously diverse microbial communities, comprising thousands of species-level operational taxonomic units (OTUs), which are often influ- enced by season, location, host health, and host genotype (28–31). Marine sponges also host complex microbial communities with diversity comparable to that of corals (32) but with associations that are generally far more stable in space and time (33). Less-diverse microbial communities are found in the sea anemone Aiptasia, where the number of OTUs is generally in the low hundreds (34). Due to the close taxonomic relationship of Aiptasia with coral and its comparatively simple microbial community, it has been proposed as a model organism for studying coral microbiology and symbiosis (34). Some marine invertebrates also include species along a continuum of microbial diversities. Ascidians, for example, have been shown to host fewer than 10 (Polycarpa aurata) or close to 500 (Didemnum sp.) microbial OTUs within their inner tunic (35). Furthermore, species with low microbial diversity such as P. aurata can exhibit high intraspecific variation, with as few as 8% of OTUs shared among individuals of the same species (35). Taken together, the data from those studies highlight the vast spectrum of associations that marine invertebrates form with microbial communities in terms of diversity, composition, and stability (Fig. 1).
While previous research has provided a good understanding of the composition of marine invertebrate microbiomes, our understanding of how the microbiome interacts with the host, and of the potential to coevolve, is far more limited. Moreover, the
FIG 1 Spectrum of microbial diversity associated with different compartments of marine invertebrates. Microbial associations may involve a single symbiont in a specialized organ or over 1,000 operational taxonomic units (OTUs) associated with tissues. The levels of OTUs reported in the figure represent the highest recorded in the referenced study for that species. Reported levels of diversity may differ significantly within the same species across different studies.
Minireview ®
January/February 2019 Volume 10 Issue 1 e02241-18 mbio.asm.org 3
D ow
nl oa
de d
fr om
h tt
ps :/
/j ou
rn al
s. as
m .o
rg /j
ou rn
al /m
bi o
on 0
2 F
eb ru
ar y
20 22
b y
24 .1
16 .2
51 .2
22 .
increasing number of studies generating tremendous volumes of host-associated microbiome sequence data requires theoretical development to interpret these rela- tionships. Coevolved microbial symbionts are presumed to be intimately linked with host fitness and metabolism (36); therefore, understanding these relationships in marine invertebrates will have direct implications for health and disease processes in these animals. Three research criteria arise for examining coevolution in marine inver- tebrates: (i) identifying stochastic and deterministic microbial components of the microbiome, (ii) assessing codivergence of host and microbe, and (iii) confirming an intimate association between host and microbe related to shared metabolic function (metabolic collaboration). While each of these criteria may be fulfilled without the involvement of coevolution (26, 37, 38), evidence of their existence in combination provides a strong basis for establishing coevolution patterns (Fig. 2). This review positions these three criteria in coevolution as representing a complementary approach to the study of complex marine invertebrate microbiomes by drawing from examples of model systems. Focussing on keystone coral reef invertebrates, this review also evaluates the current evidence for each criterion. Finally, while parasites and pathogens also contribute to host coevolution, the focus of this review is mutualistic symbionts; thus, pathogens and parasitism are not discussed.
BOX 2: GLOSSARY (i) Codivergence. Two organisms which speciate or diverge in parallel as illus-
trated by topological congruency of phylogenetic trees. (ii) Coevolution. Reciprocal adaptation of one (or more) lineage(s) in response to
another (or others). (iii) Holobiont. A host organism and its associated microbial community. (iv) Hologenome. The collective genomes of a host and its associated microbial
community, which may act as a unit of selection or at discrete levels. (v) Metabolic collaboration. Two or more oganisms that are linked through
metabolic interactions, generally to the benefit of one another. (vi) Metagenome. The collective microbial genes recovered from an environmen-
tal sample, usually predominantly prokaryotic. (vii) Metatranscriptomics. Quantification of the total microbial mRNA in a sample
as an indication of gene expression and active microbial functions. (viii) Microbiome. The total genetic make-up of a microbial community associated
with a habitat. (ix) Microbiota. The community of microorganisms residing in a particular habitat,
usually a host organism. (x) Phylosymbiosis. The rentention of a host phylogenetic signal within its
associated microbial community. (xi) Virome. The total viral genetic content recovered from an environmental
sample.
UNTANGLING PATTERNS OF HOST-MICROBE COEVOLUTION IN A WEB OF MICROBES
(i) Phylosymbiosis and neutral theory—identifying stochastic and determinis- tic components of the microbiome. Host-microbe coevolution may occur to some degree at the level of the hologenome, i.e., reciprocal evolution of the host genome and microbiome (12). Therefore, it is necessary to understand microbial community structure and population dynamics within the host environment. This may illustrate (i) that the microbiome associated with a host is structured through phylogenetically related host traits and may therefore retain a host phylogenetic signal (phylosymbiosis) and (ii) that certain microbes deviate from the expected patterns of neutral population dynamics, i.e., stochastic births and deaths and immigration. It is likely that phylosym- biosis and neutral population dynamics are linked; therefore, their potential to con- tribute to coevolution is discussed together.
Minireview ®
January/February 2019 Volume 10 Issue 1 e02241-18 mbio.asm.org 4
D ow
nl oa
de d
fr om
h tt
ps :/
/j ou
rn al
s. as
m .o
rg /j
ou rn
al /m
bi o
on 0
2 F
eb ru
ar y
20 22
b y
24 .1
16 .2
51 .2
22 .
Homocysteine + Serine (host diet & metabolism)
Cystathionine B-synthase (symbiont enzyme)
Cystathionine
Cystathionine y-lyase (host enzyme)
Cysteine
S-H CH2
C COOH H2N H
Bacteria spp. 1
Host spp. A
d)
Host phylogeny Microbial dendrogram Host species
A
B
C
D
a)
b)
Host phylogeny Microbial phylogeny
Bacteria spp. 1
A
B
C
D
Relative abundance of microbes in host sample
Fr eq
ue nc
y of
m ic
ro be
s in
h os
t Bacteria spp. 1
Bacteria spp.2 Low High
0
1
c)
FIG 2 Hypothetical scenario addressing three criteria for host-microbe coevolution in species A to D. (a) Phylosymbiosis shown through hierarchical clustering of the microbial community, resulting in a microbial dendrogram which mirrors host phylogeny. (b) Neutral model showing the expected occurrence of microbes based on neutral population dynamics (blue line). As the relative abundance increases, so too does the occurrence in host samples. The members of bacterial species group 1 (Bacteria spp. 1) are therefore more abundant than would be expected by chance and may indicate active selection, while the members of Bacteria spp. 2 are less abundant. (c) Codivergence of the members of Bacteria spp. 1 with their hosts. The members of Bacteria spp. 1 are found within the microbial community of each host species and appear to be actively selected for. Their phylogeny indicates a host split at the strain level followed by diversification within each host species. Congruence between host and microbial lineages suggests important host-microbe interactions and warrants further investigation. (d) Metabolic collaboration between the members of Host spp. A and those of Bacteria spp. 1. Fluorescence in-situ hybridization (FISH) confirms that the members of Bacteria spp. 1 are located within bacteriocyte cells in the tissues of Host spp. A. Genome and transcriptome data for each species suggest that the amino acid cysteine is produced by the activity of a metabolic pathway shared between host and microbe. In corals of the genus Acropora, for example, the genome is incomplete with respect to biosynthesis of cysteine and represents a potential pathway for collaborations of host and microbe (101). Hypothetically, the amino acids homocysteine and serine (potentially sourced from host diet and metabolism) are combined to form cystathionine through the enzyme cystathionine V synthase (provided by the host’s endosymbiont). The host enzyme cystathionine �-lyase then breaks down cystathionine to form cysteine.
Minireview ®
January/February 2019 Volume 10 Issue 1 e02241-18 mbio.asm.org 5
D ow
nl oa
de d
fr om
h tt
ps :/
/j ou
rn al
s. as
m .o
rg /j
ou rn
al /m
bi o
on 0
2 F
eb ru
ar y
20 22
b y
24 .1
16 .2
51 .2
22 .
The term “phylosymbiosis” is not intended to imply coevolution (12, 38); however, coevolution of a host and microbiome may reinforce patterns of phylosymbiosis. There are many host traits that correlate with host phylogeny, some of which can act as environmental filters, preventing the establishment of microbes in the host environ- ment. Thus, neutral population dynamics, with host traits acting as an ecological filter to microbial immigration, may be sufficient to result in phylosymbiotic patterns (39, 40). However, host traits are not static; thus, the evolution of these microbial niches may further drive the radiation of the microbes that reside within them. In turn, the continuous colonization over many generations of a microbial community likely adds to the selective pressure on host traits. Therefore, ecological filtering of microbes through host traits and coevolution of a host and microbiome need not be mutually exclusive in the appearance of phylosymbiosis (39). Moreover, assessing patterns of phylosymbiosis and neutral population dynamics also allows the detection of microbes that deviate from these patterns and may identify important microbial species that are actively selected for (or against) by the host. In this context, neutral models can simulate expected microbial abundance, allowing easier detection of microbes that do not fit these patterns (41). This reasoning justifies consideration of phylosymbiosis and microbial population dynamics in assessing coevolution in complex holobionts.
Patterns of phylosymbiosis are frequently detected in complex holobionts. One particular study tested for phylosymbiosis across 24 species of terrestrial animals from 4 groups that included Peromyscus deer mice, Drosophila flies, mosquitos, and Nasonia wasps and an additional data set of 7 hominid species (42). Since these animals (with the exception of hominids) could be reared under controlled laboratory conditions, environmental influences could be eliminated, leaving the host as the sole factor influencing the microbial community. Under these conditions, phylosymbiotic patterns were clearly observed for all five groups, with phylogenetically related taxa sharing similar microbial communities and microbial dendrograms mirroring host phylogenies. Similar patterns of phylosymbiosis have been observed in a growing number of terrestrial systems, including all five gut regions in rodents (43), the skin of ungulates (44), the distal gut in hominids (45), and roots of multiple plant phyla (46), providing evidence that such patterns are common among host-associated microbiomes.
In the marine environment, two major studies, one involving 236 colonies across 32 genera of scleractinian coral collected from the east and west coasts of Australia (47) and the other involving 804 samples of 81 sponge species collected from the Atlantic Ocean, Pacific Ocean, and Indian Ocean and the Mediterranean Sea and Red Sea (32), have provided the most convincing examples of phylosymbiosis. Both studies found a significant evolutionary signal of the host with respect to microbial diversity and composition. Specifically, mantel tests were used to delineate the finding that closely related corals and sponges hosted more extensively similar microbial communities in terms of composition than would be expected by chance. In the case of corals, the similarity was seen in the skeleton and, to a lesser extent, in the tissue microbiome, while the mucus microbiome was more highly influenced by the surrounding environ- ment (47). However, both studies found that host species was the strongest factor in explaining dissimilarity among microbial communities. Additional studies on both cold water and tropical sponges have found similar phylogenetic patterns within the microbiome of the host species (48, 49). Together, these results suggest that host phylogeny (or associated traits) has a significant role in structuring associated microbial communities, although there are additional factors related to host identity (and unre- lated to phylogeny) that also likely play a major role.
Most studies to date have focused on the microbes that adhere to these patterns of phylosymbiosis, though more-useful information arguably could be determined from the microbes that do not. Since phylosymbiosis is a pattern that shows correlations between microbiome dissimilarity and host phylogeny, it does not indicate active microbial selection or cospeciation (38), and the species that deviate from these patterns would be interesting targets for studies of codivergence and metabolic collaboration (see below). Neutral models have been applied to three species of
Minireview ®
January/February 2019 Volume 10 Issue 1 e02241-18 mbio.asm.org 6
D ow
nl oa
de d
fr om
h tt
ps :/
/j ou
rn al
s. as
m .o
rg /j
ou rn
al /m
bi o
on 0
2 F
eb ru
ar y
20 22
b y
24 .1
16 .2
51 .2
22 .
sponges, a jellyfish, and a sea anemone, and while neutral models have been shown to fit well to the expectation of microbial abundance in sponges (which also show phylosymbiosis), jellyfish and sea anemone microbiomes were found to be associated with a higher level of nonneutrality (40). Potential reasons for nonneutrality include the presence of a more sophisticated immune system in cnidarians that provides active selection on certain microbial taxa and that the microbiomes in such cases are more transient or a combination of the two. In summary, neutral population dynamics filtered through phylogenetically related host traits likely result in, or at least contribute to, the observed patterns of phylosymbiosis. This does not necessarily mean that the pattern is unimportant or is not contributing to coevolution at the hologenome level, and it may be that the communities of microbes that follow these patterns are responsible for broad ecological functions (50). On the other hand, microbes that deviate from these patterns may be responsible for more-specific functions and are of high interest to those trying to identify symbionts and coevolution at the microbial species or strain level.
(ii) Codivergence—microbial phylogeny and host phylogeny are congruent. The second criterion in assessing host-microbe coevolution is that of whether individ- ual microbial lineages and their hosts have matching phylogenies (22, 24, 51). Codi- vergence implies a tightly coupled, long-term interaction between two species and can potentially identify beneficial symbionts (or parasites) that have coevolved with the host (26). However, it is also important that codivergence can arise due to processes other than coevolution, such as one species adaptively tracking another, which would imply that the evolution is not reciprocal, or two species responding independently to the same speciation event or environmental stress (37). In known cases of coevolution, phylogenies of hosts and their microbial symbionts are congruent (16, 51, 52). However, in complex and uncharacterized systems, this strategy can be reversed to identify potential symbionts. Therefore, the main value of investigating codivergence in com- plex associations is to identify those specific microbes on which to focus further attention.
Codivergence has been demonstrated in the case of Hydra viridissima, a freshwater relative of marine cnidarians, and its photosymbiont Chlorella (53). In this system, photosynthetically fixed carbohydrates from Chlorella are transported to its host (54), and phylogenetic analysis of 6 strains of H. viridissima and their vertically transmitted symbionts revealed clear congruency of host and symbiont topologies (55). In more- complex systems, patterns of codivergence have been illustrated in the gut microbiota of hominids (25). Analysis of fecal samples from humans, wild chimpanzees, wild bonobos, and wild gorillas showed that four clades of bacteria from the dominant families Bacteroidaceae and Bifidobacteriaceae codiverged with host phylogeny. Impor- tantly, this example illustrates one possible way of identifying codivergence in complex holobionts where the symbionts are unknown. Since bacteria from the families Bacte- roidaceae, Bifidobacteriaceae, and Lachnospiraceae are known to dominate the gut of hominids, multiple primer sets targeting each individual family were utilized, and phylogenetic analyses of the families were completed independently. Furthermore, instead of using the relatively slowly diverging 16S rRNA gene, the fast-evolving and variable gene encoding DNA gyrase subunit B was used for bacterial phylogenetics. Similar methods may be applied to complex marine invertebrates such as coral and sponges, where 16S rRNA gene studies have identified prominent bacteria.
Within complex marine invertebrate holobionts, codivergence has been most clearly demonstrated in cold-water sponges in the family Latrunculiidae. The microbiomes of six species within this family were dominated by a single betaproteobacterial OTU, and the phylogeny of this OTU was highly congruent with that of the host (56). Further- more, gene expression analysis suggested that the dominant betaproteobacteria are active members of the microbiome rather than dormant or nonviable members; however, whether or not this potential symbiont and its host participate in metabolic collaboration is unknown, highlighting an example warranting further investigation. The microbiomes of many other marine invertebrates are dominated by members of
Minireview ®
January/February 2019 Volume 10 Issue 1 e02241-18 mbio.asm.org 7
D ow
nl oa
de d
fr om
h tt
ps :/
/j ou
rn al
s. as
m .o
rg /j
ou rn
al /m
bi o
on 0
2 F
eb ru
ar y
20 22
b y
24 .1
16 .2
51 .2
22 .
the genus Endozoicomonas (57). A pan-genomic analysis of the
Collepals.com Plagiarism Free Papers
Are you looking for custom essay writing service or even dissertation writing services? Just request for our write my paper service, and we'll match you with the best essay writer in your subject! With an exceptional team of professional academic experts in a wide range of subjects, we can guarantee you an unrivaled quality of custom-written papers.
Get ZERO PLAGIARISM, HUMAN WRITTEN ESSAYS
Why Hire Collepals.com writers to do your paper?
Quality- We are experienced and have access to ample research materials.
We write plagiarism Free Content
Confidential- We never share or sell your personal information to third parties.
Support-Chat with us today! We are always waiting to answer all your questions.
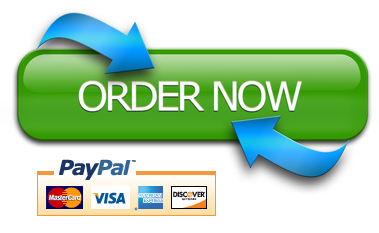