How is serum calcium regulated? 1.??????What body system dysfunctions would cause calcium abnormalities? 2.??????The GI tract
CAT1
Chapters 240 and 241 in your Principles and Practice of Hospital Medicine text detail several electrolyte abnormalities. After completing your assigned readings, answer the following questions;
1. How is serum calcium regulated?
1. What body system dysfunctions would cause calcium abnormalities?
2. The GI tract, kidneys, and skeletal system are integral in regulating calcium.
2. What are potential causes of hypercalcemia? How can you differentiate the cause of hypercalcemia in your patient?
3. How is hypercalcemia diagnosed and managed?
4. What are potential causes of hypocalcemia?
5. What are potential causes of potassium and magnesium disorders?
1. What is pseudohypokalemia? What is the treatment for this?
6. What are the potential consequences of potassium and magnesium disorders?
7. How are potassium and magnesium disorders treated?
1. What is the function of Calcium Gluconate in lowering potassium?
8. How are potassium disorders treated in clinical situations with rapid potassium shifts, such as diabetic ketoacidosis, hyperglycemic hyperosmolar state, and periodic paralysis?
1578185 – McGraw-Hill Professional ©
hanges are present. It has become less common with the increased use of plasma for potassium measurement, rather than serum. Plasma is the supernatant collected from heparinized whole blood, whereas serum is the supernatant remaining after centrifugation of a clotted whole blood sample. Serum K+ is normally 0.3 mmol/L above the plasma K+, but may be higher if K+ is released from the clot formed in the tube. If pseudohyperkalemia is suspected, the plasma potassium should be measured instead of the serum potassium. Pseudohyperkalemia can also occur due to potassium release from muscle cells following prolonged constriction with a tourniquet or limb exercise while a tourniquet is in place.
Redistribution of potassium
Redistribution of potassium from the intracellular to the extracellular space can occur in severe acidosis due to nonorganic acid metabolic acidosis, hyperosmolar states, tissue breakdown, and hyperkalemic periodic paralysis. Organic acids such as lactic acid and ketoacids are less likely to cause hyperkalemia than non-organic acids. These organic acids have greater transmembrane mobility, allowing movement into cells with H+, rather than movement of K+ out of cells in exchange for H+. Hyperkalemia in diabetic ketoacidosis usually results from insulin deficiency and hyperosmolality, rather than acidosis. Hyperglycemia increases extracellular osmolality, drawing water from cells down the osmotic gradient. Potassium follows the water movement (solvent drag), and hyperkalemia results.
Tissue breakdown may liberate large amounts of intracellular potassium, resulting in rapid, life-threatening increases in extracellular potassium. Rhabdomyolysis, tissue necrosis, tumor lysis with chemotherapy, and large hematomas are common causes. In rhabdomyolysis, hypokalemia may precede hyperkalemia, and contribute to muscle breakdown by causing vasoconstriction and decreased blood flow to the involved muscle.
Hyperkalemic periodic paralysis is an autosomal dominant disorder involving the muscle cell sodium channel. During these episodes, potassium moves from the intracellular to extracellular space, accompanied by movement of sodium and water into the cell. The hyperkalemia is accompanied by transient weakness or paralysis.
Decreased potassium excretion
Potassium excretion in the distal nephron depends upon adequate urine flow, aldosterone, and activity of the basolateral Na+/K+-ATPase. The reabsorption of luminal Na+ through aldosterone-sensitive epithelial sodium channels (ENaCs) creates an electrochemical gradient for K+ excretion in the urine. Hyperkalemia can occur in aldosterone-resistant or deficient states by attenuating the electrochemical gradient for K+ secretion. Acquired mineralocorticoid resistance from diabetes mellitus, obstructive uropathy, chronic tubulointerstitial disease, sickle cell anemia, lupus nephritis, and medications, as well as decreased mineralocorticoid production (including medication induced), are commonly associated with type IV renal tubular acidosis (RTA). Type IV RTA is characterized by hyperkalemia, normal anion gap hyperchloremic acidosis, with serum HCO3 values ranging between 16 and 22 mmol/L, and decreased urinary NH4
+ production, causing a positive urine anion gap [Urine (Na+) + (K+) – (Cl−)]. In acute and chronic tubular injury, a
1578185 – McGraw-Hill Professional ©
decreased responsiveness to aldosterone can contribute to hyperkalemia. For acute kidney injury associated with prerenal azotemia or hypovolemic states, decreased urinary flow to the distal tubule and a reduction in sodium-potassium exchange can contribute to hyperkalemia.
Excessive potassium intake
Excessive intake of K+ rarely causes hyperkalemia in the setting of normal renal function. Renal secretion of potassium is typically adequate at glomerular filtration rates (GFR) above 20 to 30 mL/min/1.73 m2. Patients with end-stage renal disease (ESRD) on hemodialysis usually tolerate a daily K+ intake of 2000 mg (51 mEq). Upregulated gut potassium excretion and shifts in transcellular K+ prevent hyperkalemia between dialysis sessions. The loss of these mechanisms may result in the rapid development of hyperkalemia, especially with large exogenous loads of potassium, such as massive blood transfusions. Irradiation of blood and increased age of the blood increase the amount of free potassium that is released during the blood transfusion. Seven-day-old blood has approximately 23 mmol/L of K+, while 42-day-old blood has approximately 50 mmol/L of K+.
PRACTICE POINT
In the setting of acute kidney injury, hyperkalemia associated with rapid transcellular potassium shifts from the intracellular to extracellular compartment can be seen with rhabdomyolysis, tissue necrosis, tumor lysis, and large hematomas. Hyperkalemia in this setting may progress rapidly to cause life-threatening arrhythmias. Emergent nephrology consultation for possible dialysis is required.
SIGNS AND SYMPTOMS
Clinical effects of hyperkalemia relate to altered membrane excitability due to changes in the transcellular potassium gradient. Severe hyperkalemia leads to cardiac arrhythmias and conduction abnormalities. It may also cause weakness of the lower extremities, progressing superiorly to cause flaccid paralysis and respiratory failure. This presentation may mimic Guillain-Barré syndrome, but is easily differentiated by the response to potassium correction. Hyperkalemia may also contribute to metabolic acidosis by interfering with renal ammonium excretion.
EVALUATION OF HYPERKALEMIA IN THE HOSPITALIZED PATIENT
In the hospital setting, the initial evaluation of hyperkalemia includes monitoring for life- threatening arrhythmias, checking for pseudohyperkalemia, eliminating exogenous sources of potassium, evaluating renal function, and evaluating for rapid transcellular shifts of potassium (Figure 241-1).
1578185 – McGraw-Hill Professional ©
Figure 241-1 Diagnostic assessment of hyperkalemia. (ACE, angiotensin-converting enzyme inhibitor; ARB, angiotensin receptor blocker; DKA, diabetic ketoacidosis; ECG, electrocardiogram; GFR, glomerular filtration rate; HHS, hyperosmolar hyperglycemic state; NSAIDs, nonsteroidal anti-inflammatory drugs.)
ECG changes
Even mildly elevated K+ levels may be associated with ECG changes, especially in the setting of rapid rises in plasma K+ values. If ECG changes from hyperkalemia are found or if the plasma K+ value is ≥ 7.0, continuous telemetry is indicated and should continue until the plasma K+ value is ≤ 5.8 and there are no hyperkalemic ECG changes. Frequent chemistry checks are indicated for plasma K+ greater than 5.8 or for hyperkalemia associated with ECG changes, severe hyperglycemia, or any clinical condition which predisposes the patient to rapid changes in plasma K+.
1578185 – McGraw-Hill Professional ©
Plasma potassium levels do not correlate with specific ECG changes. A symmetric increase in T wave height may be seen initially. Hyperkalemia-induced peaked T waves may be difficult to distinguish from the hyperacute T waves of myocardial injury. As potassium levels rise further, flattening of the P waves, prolongation of the PR interval, and prolonged QRS duration occur. Eventually, atrial standstill and a sine wave ECG pattern may be seen (Figure 241-2).
Figure 241-2 ECG changes associated with hyperkalemia and hypokalemia. (Reproduced, with permission, from Flomenbaum N, Goldfrank LR, Hoffman R, et al. eds. Goldfrank’s Toxicologic Emergencies, 8th ed. New York, NY: McGraw-Hill; 2006. Fig. 5-11.)
Urine potassium, transtubular potassium gradient, and urine potassium/creatinine
A 24-hour urine K+ measurement can help differentiate between renal and nonrenal causes of hyperkalemia. With hyperkalemia, urinary excretion of K+ should exceed 40 mmol/d.
1578185 – McGraw-Hill Professional ©
Alternatively, the transtubular potassium gradient (TTKG), a measurement of potassium secretion by the distal nephron corrected for urine osmolality, has been used:
TTKG = (KU/KS) × (SOsm/UOsm)
KU and KS are the concentrations of K + in the urine and serum, and SOsm and UOsm are
the osmolalities of the serum and urine, respectively. Plasma potassium and osmolality can be used instead of serum values to estimate TTKG with minimal effect on clinical interpretation. The accuracy of the TTKG has been called into question by recent studies of urea and potassium handling in the renal tubule. Previous studies using TTKG have suggested that patients with normal renal function and normal potassium intake have a TTKG of 8 to 9. In hyperkalemia, a low TTKG (< 5-7) suggests an inappropriately low secretion of potassium. A high TTKG with hyperkalemia suggests normal aldosterone action and an extrarenal cause of hyperkalemia, except in cases of volume depletion where aldosterone secretion is enhanced with a TTKG > 7, but total renal potassium excretion is limited by low urine flow.
When the TTKG is inappropriately low in the setting of hyperkalemia, an increase in the TTKG to >10 hours after the administration of 0.05 mg of fludrocortisone suggests hypoaldosteronism. If fludrocortisone has no effect on the TTKG, drug-induced or intrinsic renal resistance to aldosterone are likely.
If further studies cast doubt on the usefulness of TTKG, the urine potassium/creatinine ratio will likely be used more frequently when a 24-hour urine potassium is not available. It has been suggested that a patient with hyperkalemia and a normal renal response should have a spot urine ratio of >200 mmol K+/g creatinine (=22.6 mmol K+/mmol creatinine). For a typical patient with a daily creatinine excretion of over 1 g/d, this is significantly more than the 24-hour urinary K+ excretion of >40 mmol used by some clinicians as a cutoff for adequate renal potassium clearance in the setting of hyperkalemia. This further underscores the point that it is difficult to define an exact cutoff for expected renal potassium excretion or urine K+/Cr in the face of hyperkalemia without additional investigation.
TREATMENT OF HYPERKALEMIA
Treatments exist for hyperkalemia which cause net excretion or removal of potassium, such as gastrointestinal resins and laxatives or hemodialysis. As these therapies may not act immediately or may involve logistical difficulties, they are often used in conjunction with short-acting temporizing measures, such as cardiac membrane stabilization with calcium, and agents such as insulin that cause transcellular potassium shifts (Table 241- 2).
TABLE 241-2 Treatment of Hyperkalemia
Mechanism Treatment Dose Onset Duration Comments Cardiac membrane stabilization
Calcium Calcium gluconate 10 mL of 10% solution infused over 2-3 min.
1-3 min 30-60 min
Do not mix with bicarbonate; extreme caution if on digoxin; calcium chloride is an alternative, but poses a risk of tissue
1578185 – McGraw-Hill Professional ©
necrosis, and requires a central line
Redistribution Insulin 10 units regular insulin IV. If BG < 250 mg/dL, give 50 mL of 50% dextrose.
10-20 min 4-6 h peak 30- 60 min
Most reliable treatment to induce redistribution
Redistribution Beta-2 agonist
Albuterol 10- 20 mg nebulized.
30 min 2-4 h peak 90 min
Dose is significantly higher than dose for respiratory treatments; use with caution in patients at risk for side effects such as tachycardia and myocardial ischemia
Removal Kayexalate 30-60 g oral in 20% sorbitol or 60 grams in 250 mL water by retention enema.
1-2 h Variable Risk of colonic necrosis if used in postoperative patients; do not use sorbitol formulation when administering via enema
Removal Hemodialysis — Immediate Same as dialysis duration
Intermittent or continuous
Cardiac membrane stabilization
Intravenous calcium stabilizes the cardiac membrane by inhibiting membrane depolarization. It is the most important initial treatment for severe hyperkalemia. Two forms of calcium are commonly available: calcium gluconate and calcium chloride. Calcium gluconate is preferred because it can be administered through a peripheral intravenous line, whereas calcium chloride requires a central venous line to prevent tissue necrosis. Tissue necrosis can occur if calcium chloride leaks from the venous access into the surrounding tissue. A 10 mL ampule of calcium gluconate contains 90 mg (2.3 mmol) of elemental calcium, and a 10 mL ampule of calcium chloride contains 272 mg (7.0 mmol) of elemental calcium.
The initial dose of calcium gluconate is 10 mL of 10% solution infused over 2 to 3 minutes. An equivalent amount of elemental calcium is contained in 3.3 mL of 10% calcium chloride. The onset of action is 1 to 3 minutes, and duration of action is 30 to 60 minutes. Calcium cannot be mixed with bicarbonate solutions, because precipitation of CaCO3 occurs.
1578185 – McGraw-Hill Professional ©
3 Administration of intravenous calcium to patients taking digoxin requires extreme
caution, as calcium has been shown to potentiate the effects of digoxin toxicity in animal models, especially at very rapid infusion rates. The risk of calcium administration in this setting can be reduced by infusing the calcium gluconate over 20 to 30 minutes. Another option is to administer digoxin immune fab to neutralize the effect of the digoxin.
PRACTICE POINT
In the setting of digoxin toxicity, mild hyperkalemia (serum K+ > 5.0 mmol/L) has been linked to significant mortality, and digoxin immune fab may be indicated.
Potassium redistribution
Insulin is the most reliable means of inducing transcellular potassium shifts. The usual dose is 10 units of regular insulin intravenously, followed immediately by 50 mL of 50% dextrose (25 g of dextrose). For a blood glucose > 250, insulin can be administered alone with close glucose monitoring. The effect begins in 10 to 20 minutes, peaks at 30 to 60 minutes, and lasts 4 to 6 hours. Potassium levels typically drop by 0.5 to 1.2 mmol/L. An infusion of 10 units of regular insulin can also be administered over 1 hour in 10% dextrose.
Beta-2 agonists have an additive effect with insulin in transiently reducing plasma potassium by redistribution. High doses of nebulized albuterol are used, typically 10 to 20 mg of nebulized albuterol in 4 mL of normal saline over 10 minutes. Plasma potassium levels usually fall by 0.5 to 1 mmol/L. The effect begins in 30 minutes, peaks at 90 minutes, and lasts 2 to 4 hours. Intravenous albuterol has also been used, but it is not available in the United States. As some patients, including those with renal failure, have a reduced response to albuterol, it should not be the only agent used. Caution should be exercised for individuals at risk for side effects such as cardiac ischemia from the resulting increase in heart rate.
Sodium bicarbonate (NaHCO3) does not reliably lead to the redistribution of potassium, and it should not be considered as first-line therapy for hyperkalemia. This is especially true in high anion gap acidosis, where hyperkalemia is usually not a direct consequence of the presence of organic acids. If intravenous or oral NaHCO3 is used to treat metabolic acidosis caused by nonorganic anions, which is usually associated with a normal anion gap, plasma potassium may fall, but it is not preferred treatment even in this setting. Oral NaHCO3 is useful for chronic treatment of type IV renal tubular acidosis.
Potassium removal
Sodium polystyrene sulfonate (Kayexalate) exchanges Na+ for K+ in the gastrointestinal tract. When taken orally, sorbitol has been typically added to the resin to speed passage through the gastrointestinal tract. However, an FDA warning has been issued about the risk of colonic necrosis when kayexalate is used with sorbitol, and thus the powdered form of kayexalate not premixed with sorbitol is preferred. If Kayexalate with sorbitol is the only form available, diluting with water is appropriate. Each gram of resin binds 0.5 to 1.2 mmol of K+. Oral doses of 15 to 60 g are typical. Sodium polystyrene sulfonate can also be administered rectally as a retention enema, at a dose of 30 to 60 g in 250 mL of water
1578185 – McGraw-Hill Professional ©
every 6 hours. The solution should be introduced by gravity, flushed with an additional 50 to 100 mL of nonsodium containing fluid, retained 30 to 60 minutes or longer, and cleansed with 250 to 1000 mL of nonsodium containing solution at body temperature. Sorbitol should not be used rectally due to risk of colonic necrosis. Oral or rectal Kayexalate should not be used in postoperative patients for the same reason.
Favorable data have been published for two novel potassium reduction agents. Sodium zirconium cyclosilicate (ZS-9) is a highly selective potassium trap, whereas patiromer is a nonabsorbed polymer which exchanges calcium for potassium, primarily in the distal colon. Patiromer is FDA approved, ZS-9 is under FDA review, and further studies are needed to evaluate their suitability for acute potassium reduction. Initial studies have focused on their use for more chronic potassium reduction, which may allow broader use of potassium-sparing medications.
Dialysis is required for treatment of refractory hyperkalemia. Intermittent hemodialysis removes potassium most rapidly. Continuous renal replacement therapy is an option for patients who have ongoing causes of severe hyperkalemia, such as tissue necrosis or rhabdomyolysis. Peritoneal dialysis provides gradual removal of potassium. Although peritoneal dialysis is rarely used in developed countries when the other modalities are available, peritoneal dialysis is an established therapy for acute kidney injury. Since electricity is not required for its use and access is placed in the peritoneal cavity rather than in large veins, it is an option for hyperkalemia treatment in a variety of situations such as difficulty with placing vascular access for hemodialysis, disasters associated with overwhelming caseloads, or power failure
Diuretics are unreliable for the acute treatment of hyperkalemia in the setting of compromised renal function. In patients with adequate renal function, the combination of a loop and thiazide diuretic is more effective for potassium removal than either alone. Of the loop diuretics, torsemide and bumetanide have higher bioavailability than furosemide.
Potassium intake reduction
The typical daily potassium intake for a patient with end-stage renal disease is 2000 mg (51 mmol) of potassium per day, but patients with acute kidney injury may require even more stringent potassium restriction.
HYPOKALEMIA ETIOLOGY
Hypokalemia (K+ < 3.5 mmol/L) can result from redistribution, increased potassium excretion from renal and nonrenal sources, or decreased potassium intake. Medications, especially loop and thiazide diuretics, frequently cause hypokalemia. Hypokalemia is common with tubular toxins such as amphotericin B and cisplatin. High doses of penicillin or semisynthetic penicillins such as ticarcillin and carbenicillin occasionally cause renal potassium wasting in the distal nephron due to a transient anion effect. Inhalation of toluene (glue sniffing) may cause distal renal tubular acidosis with associated hypokalemia.
Pseudohypokalemia
1578185 – McGraw-Hill Professional ©
Falsely low serum or plasma potassium values may occur in conditions such as acute leukemia due to time-dependent uptake of potassium by the increased abnormal white cell mass. Rapid analysis of the sample, or storing the sample at 4°C prior to analysis, can prevent the potassium uptake and confirm the diagnosis of pseudohypokalemia.
Redistribution
Insulin directly stimulates potassium entry into cells by increasing the activity of the Na+/K+-ATPase pump. This mechanism is independent of stimulation of cellular glucose entry. Beta-2-adrenergic activators stimulate Na+/K+-ATPase mediated cellular potassium uptake by a slightly different cellular mechanism. Thus, insulin and beta-2-adrenergic activation may act synergistically to cause hypokalemia. Aldosterone stimulates direct cellular uptake and redistribution due to increased Na+/K+-ATPase activity. It also acts in the distal renal tubule to enhance potassium excretion.
Thyrotoxic periodic paralysis causes severe hypokalemia by redistribution, in conjunction with extremity and limb girdle weakness, hypophosphatemia, and hypomagnesemia. It is more common in patients of Asian and Hispanic origin. Attacks often occur during rest after vigorous physical activity, and can also be precipitated by carbohydrate-rich meals. Other rare genetic forms of hypokalemic periodic paralysis also exist.
Nonrenal potassium losses
Common nonrenal causes of hypokalemia include intestinal loss of potassium from diarrhea, celiac disease, ileostomy, and chronic laxative abuse. Potassium loss from vomiting and nasogastric suctioning can result in hypokalemia, although renal potassium losses from aldosterone activation may be more important in this setting. Potassium losses through the skin are usually low, except in the setting of extreme physical exertion. Severe burns may lead to hypokalemia by multiple mechanisms.
Renal potassium loss
Aldosterone-producing adenomas (Conn syndrome) cause hypertension and hypokalemia by stimulation of aldosterone receptors in the distal renal tubule. Cortisol also activates the aldosterone receptor. Normally, cortisol is converted to cortisone by 11-beta- hydroxysteroid dehydrogenase-2 (11βHSD-2) before it can reach the aldosterone receptor. Very high cortisol levels, as seen in Cushing syndrome, overwhelm the ability of 11βHSD-2 to degrade cortisol to cortisone, and the nondegraded cortisol activates the aldosterone receptor in the distal tubule and precipitates hypokalemia. Similarly, glycyrrhizinic acid, a component of black licorice that is sometimes added to chewing tobacco, inhibits 11βHSD-2, preventing cortisol degradation and causing hypertension and hypokalemia. With vomiting and nasogastric suctioning, potassium wasting in the urine occurs through aldosterone secretion in the setting of volume depletion, as noted above.
Hypokalemia can be associated with an RTA, due to disease of either the proximal (type II RTA) or distal (type I RTA) renal tubules. Either can result from autoimmune, genetic, endocrine, medication-induced, toxin-induced, or idiopathic causes. Rare causes of hypokalemia associated with metabolic alkalosis involve defects in the thick ascending limb of Henle transport proteins (Bartter syndrome), mutations in the thiazide-sensitive
1578185 – McGraw-Hill Professional ©
Na+/Cl− cotransporter in the distal convoluted tubule (Gitelman syndrome), or increased activation of the epithelial sodium channel (ENaC) in the distal tubule (Liddle syndrome).
Decreased potassium intake
Decreased potassium intake is rarely a cause of hypokalemia, except in severe malnutrition. For patients with normal renal function who consume the recommended 4700 mg (120 mmol) of potassium per day, 90% (108 mmol) is excreted in the urine. When intake is sharply reduced, urinary potassium loss decreases to < 15 to 20 mmol/d in order to conserve potassium.
SIGNS AND SYMPTOMS
Individuals with serum potassium levels between 3.0 and 3.5 mEq/L are often asymptomatic. However, there is a risk of cardiac arrhythmias for individuals with predisposing conditions such as coronary artery disease. At serum potassium levels between 2.5 and 3.0 mEq/L, patients report generalized weakness and constipation. When serum potassium levels drop below 2.5 mEq/L, there is an increased risk of muscle necrosis and rhabdomyolysis. At serum potassium levels less than 2.0 mEq/L, ascending paralysis and respiratory failure can occur.
EVALUATION OF HYPOKALEMIA
An algorithm for the diagnosis of hypokalemia is presented in Figure 241-3. Pseudohypokalemia and transcellular shifts should be excluded. Magnesium levels should be measured early in the workup of hypokalemia. Many disorders, such as diarrhea and excess diuresis, deplete both potassium and magnesium. Moreover, hypomagnesemia may lead to renal potassium wasting via potassium channels in the distal tubule, and make hypokalemia more refractory to treatment.
1578185 – McGraw-Hill Professional ©
Figure 241-3 Diagnostic assessment of hypokalemia. (DKA, diabetic ketoacidosis; RTA, renal tubular acidosis.)
1578185 – McGraw-Hill Professional ©
The next step is determining whether hypokalemia arose from a renal or nonrenal source. An obvious cause may be apparent, such as profuse diarrhea or escalating doses of a loop or thiazide diuretic. When the cause of hypokalemia is unclear, a 24-hour urine potassium measurement may differentiate between renal and nonrenal losses. In the setting of hypokalemia, urinary K+ losses should fall to less than 15 to 20 mmol/d. Potassium excretion above this level suggests a renal contribution to hypokalemia.
When a 24-hour urine K+ is not available, a spot urine ratio of 22 mmol K+/g creatinine (=2.5 mmol K+/mmol creatinine) marks the cutoff between hypokalemia secondary to intracellular shifts (if < 22 mmol K+/g Cr) and renal loss (if >22 mmol K+/g creatinine).
The TTKG (see above) has been used to help establish the cause of hypokalemia, but the use of TTKG has been cast into doubt by recent studies of urea and K+ handling in the renal tubule. An inappropriately high TTKG (> 4) in hypokalemia has been interpreted as suggesting an increased distal potassium secretion and renal potassium loss. A low TTKG can occur with nonrenal potassium wasting, with urinary potassium losses from osmotic diuresis, with hypokalemia secondary to diuretics which were discontinued at the time of TTKG measurement, or with hypokalemia associated with K+ shifts.
Patients with renal potassium wasting should be further classified by acid-base status. Patients with acidosis may have RTA, diabetic ketoacidosis, or tubular dysfunction from drugs such as amphotericin B or acetazolamide. Patients with alkalosis and hypertension may have mineralocorticoid excess or Liddle syndrome. Hypokalemia with alkalosis and normal or low blood pressure may be caused by vomiting, diuretics, and Bartter or Gitelman syndrome. The spot urine chloride is a useful diagnostic tool in evaluating the etiology of hypokalemia in the setting of metabolic alkalosis, with a spot low urine chloride (< 10 mmol/L) suggesting volume depletion and a chloride-responsive state.
ECG changes
ECG changes in hypokalemia are shown in Figure 241-2. U waves appear following the T waves, and become progressively more prominent in comparison to the T waves as potassium levels decrease. Ultimately, the U wave merges with the T wave, and the QT interval appears prolonged.
TREATMENT OF HYPOKALEMIA
Potassium repletion is the cornerstone of therapy for hypokalemia. As extracellular potassium comprises a fraction of the total body potassium store, relatively large amounts of potassium are required to correct the total body potassium deficit. A plasma K+ 1 mmol/L below normal corresponds to a total body potassium deficit of approximately 200 to 400 mmol, and a drop in plasma K+ to 2 mmol/L below normal requires 400 to 800 mmol for repletion. Typically, daily repletion is significantly less than the total body deficit as the time required for redistribution is prolonged. Underlying disorders such as metabolic alkalosis that are causing or perpetuating hypokalemia must also be addressed. Continuous telemetry and frequent electrolyte checks should be performed in severe hypokalemia, or in conditions in which the serum potassium may decline rapidly, such as diabetic ketoacidosis (DKA) and hyperosmolar hyperglycemic state (HHS).
Oral repletion
1578185 – McGraw-Hill Professional ©
If there is no immediate threat to life, oral potassium can be used to treat hypokalemia. Generally, potassium chloride (KCl) is indicated for hypokalemia associated with diuretic use or volume depletion. A typical initial dose in a patient with normal renal function is 40 to 100 mmol (40-100 mEq) per day, in two to three divided doses. Liquid, wax matrix, and microencapsulated forms exist. Compliance is poor with the liquid form due to the strong taste. Although the wax matrix form is easier to swallow, it has been associated with erosions of the gastrointestinal tract. The microencapsulated formulation is associated with the fewest complications.
Other potassium preparations are available for different indications. Oral potassium phosphate is found in many foods, and is indicated for combined potassium and phosphorus depletion. Terminology may be misleading. For example, Neutra-Phos actually has more potassium than K-Phos. Potassium bicarbonate is useful for the treatment of both metabolic acidosis and hypokalemia, while potassium citrate may prevent renal stones.
Intravenous repletion
KCl is preferred for intravenous repletion. Potassium phosphate may be used for dual phosphorus and potassium depletion. Potassium can be infused through a peripheral intravenous line at a maximum rate of 10 mmol/h. Higher rates require a central venous line and continuous ECG monitoring. Infusion rates of 20 to 40 mmol/h are reserved for cases of life-threatening hypokalemia requiring emergent correction. One liter bags of IV fluids typically have a maximum of 60 mEq of K+ added in order to avoid infusing an excess amount of K+.
Intravenous potassium is a common cause of iatrogenic hyperkalemia. A typical intravenous dose with normal renal function is 20 to 40 mmol (20-40 mEq). Although 20 mmol of intravenous KCl might increase plasma K+ by 0.25 mmol/L, transcellular shifts make it difficult to predict the effect of therapy. Renal potassium clearance …
,
1578185 – McGraw-Hill Professional ©
CHAPTER 240 Calcium Disorders
Elizabeth H. Holt, MD, PhD
John P. Bilezikian, MD
Key Clinical Questions
How is serum calcium regulated? What are the causes of hypercalcemia in hospitalized patients? How is hypercalcemia diagnosed and managed? What causes hypocalcemia in hospitalized patients? How is it diagnosed and managed?
INTRODUCTION Abnormalities of calcium metabolism are common in hospital practice. Hypercalcemia has a prevalence of 0.1% in the general population and 1% among hospitalized patients. In the inpatient setting, hypercalcemia often portends serious illness, especially malignancy. Hypocalcemia is also common in the hospital, especially in patients with chronic renal failure or sepsis. Hypocalcemia may also be a manifestation of vitamin D deficiency, which has a prevalence of up to 80% on specialized geriatric inpatient units.
1578185 – McGraw-Hill Professional ©
CALCIUM METABOLISM Precise regulation of calcium homeostasis is essential because of the critical role of calcium in many physiological activities. It is the major mineral of bone. It also plays major roles in neuronal transmission, muscle contraction, and blood coagulation. Calcium is also required for the proper functioning of many enzymes, endocrine secretory processes, and biochemical signaling pathways.
NORMAL SERUM CALCIUM LEVELS
A typical laboratory range for serum total calcium concentration is between 8.4 and 10.2 mg/dL. Approximately half of this total am
Collepals.com Plagiarism Free Papers
Are you looking for custom essay writing service or even dissertation writing services? Just request for our write my paper service, and we'll match you with the best essay writer in your subject! With an exceptional team of professional academic experts in a wide range of subjects, we can guarantee you an unrivaled quality of custom-written papers.
Get ZERO PLAGIARISM, HUMAN WRITTEN ESSAYS
Why Hire Collepals.com writers to do your paper?
Quality- We are experienced and have access to ample research materials.
We write plagiarism Free Content
Confidential- We never share or sell your personal information to third parties.
Support-Chat with us today! We are always waiting to answer all your questions.
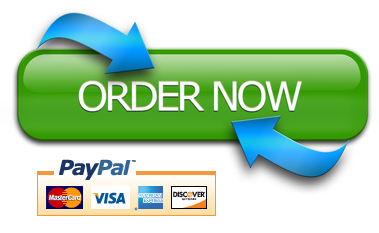